Mba1, a novel component of the mitochondrial protein export machinery of the yeast Saccharomyces cerevisiae
- PMID: 11381092
- PMCID: PMC2174334
- DOI: 10.1083/jcb.153.5.1085
Mba1, a novel component of the mitochondrial protein export machinery of the yeast Saccharomyces cerevisiae
Abstract
The biogenesis of mitochondria requires the integration of many proteins into the inner membrane from the matrix side. The inner membrane protein Oxa1 plays an important role in this process. We identified Mba1 as a second mitochondrial component that is required for efficient protein insertion. Like Oxa1, Mba1 specifically interacts both with mitochondrial translation products and with conservatively sorted, nuclear-encoded proteins during their integration into the inner membrane. Oxa1 and Mba1 overlap in function and substrate specificity, but both can act independently of each other. We conclude that Mba1 is part of the mitochondrial protein export machinery and represents the first component of a novel Oxa1-independent insertion pathway into the mitochondrial inner membrane.
Figures
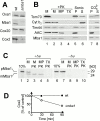
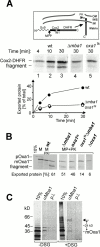
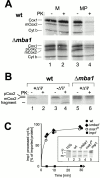
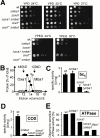
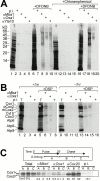
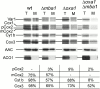
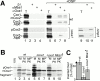
Similar articles
-
Mba1, a membrane-associated ribosome receptor in mitochondria.EMBO J. 2006 Apr 19;25(8):1603-10. doi: 10.1038/sj.emboj.7601070. Epub 2006 Apr 6. EMBO J. 2006. PMID: 16601683 Free PMC article.
-
Insertion of proteins into the inner membrane of mitochondria: the role of the Oxa1 complex.Biochim Biophys Acta. 2002 Sep 2;1592(1):79-87. doi: 10.1016/s0167-4889(02)00266-5. Biochim Biophys Acta. 2002. PMID: 12191770 Review.
-
Conserved mechanism of Oxa1 insertion into the mitochondrial inner membrane.J Mol Biol. 2005 Dec 2;354(3):520-8. doi: 10.1016/j.jmb.2005.09.054. Epub 2005 Oct 5. J Mol Biol. 2005. PMID: 16253275
-
The inner-mitochondrial distribution of Oxa1 depends on the growth conditions and on the availability of substrates.Mol Biol Cell. 2012 Jun;23(12):2292-301. doi: 10.1091/mbc.E11-06-0538. Epub 2012 Apr 18. Mol Biol Cell. 2012. PMID: 22513091 Free PMC article.
-
Protein insertion into the inner membrane of mitochondria.IUBMB Life. 2003 Apr-May;55(4-5):219-25. doi: 10.1080/1521654031000123349. IUBMB Life. 2003. PMID: 12880202 Review.
Cited by
-
The role of Coa2 in hemylation of yeast Cox1 revealed by its genetic interaction with Cox10.Mol Cell Biol. 2010 Jan;30(1):172-85. doi: 10.1128/MCB.00869-09. Mol Cell Biol. 2010. PMID: 19841065 Free PMC article.
-
Roles of Oxa1-related inner-membrane translocases in assembly of respiratory chain complexes.Biochim Biophys Acta. 2009 Jan;1793(1):60-70. doi: 10.1016/j.bbamcr.2008.05.004. Epub 2008 May 15. Biochim Biophys Acta. 2009. PMID: 18522806 Free PMC article. Review.
-
Arabidopsis thaliana Oxa proteins locate to mitochondria and fulfill essential roles during embryo development.Planta. 2013 Feb;237(2):573-88. doi: 10.1007/s00425-012-1793-9. Epub 2012 Nov 21. Planta. 2013. PMID: 23179441
-
Mdm38 interacts with ribosomes and is a component of the mitochondrial protein export machinery.J Cell Biol. 2006 Feb 13;172(4):553-64. doi: 10.1083/jcb.200505060. J Cell Biol. 2006. PMID: 16476776 Free PMC article.
-
Architecture of the large subunit of the mammalian mitochondrial ribosome.Nature. 2014 Jan 23;505(7484):515-9. doi: 10.1038/nature12890. Epub 2013 Dec 22. Nature. 2014. PMID: 24362565
References
-
- Ackerman S.H., Tzagoloff A. ATP10, a yeast nuclear gene required for the assembly of the mitochondrial F1-F0 complex. J. Biol. Chem. 1990;265:9952–9959. - PubMed
-
- Bauer M., Behrens M., Esser K., Michaelis G., Pratje E. PET1402, a nuclear gene required for proteolytic processing of cytochrome oxidase subunit 2 in yeast. Mol. Gen. Genet. 1994;245:272–278. - PubMed
-
- Bonnefoy N., Chalvet F., Hamel P., Slominski P.P., Dujardin G. OXA1, a Saccharomyces cerevisiae nuclear gene whose sequence is conserved from prokaryotes to eukaryotes controls cytochrome oxidase biogenesis. J. Mol. Biol. 1994;239:201–212. - PubMed
-
- Borst P., Grivell L.A. The mitochondrial genome of yeast. Cell. 1978;15:705–723. - PubMed
Publication types
MeSH terms
Substances
LinkOut - more resources
Full Text Sources
Molecular Biology Databases