Long-term potentiation of intrinsic excitability at the mossy fiber-granule cell synapse of rat cerebellum
- PMID: 10884304
- PMCID: PMC6772314
- DOI: 10.1523/JNEUROSCI.20-14-05208.2000
Long-term potentiation of intrinsic excitability at the mossy fiber-granule cell synapse of rat cerebellum
Abstract
Synaptic activity can induce persistent modifications in the way a neuron reacts to subsequent inputs by changing either synaptic efficacy or intrinsic excitability. After high-frequency synaptic stimulation, long-term potentiation (LTP) of synaptic efficacy is commonly observed at hippocampal synapses (Bliss and Collingridge, 1993), and potentiation of intrinsic excitability has recently been reported in cerebellar deep nuclear neurons (Aizenmann and Linden, 2000). However, the potential coexistence of these two aspects of plasticity remained unclear. In this paper we have investigated the effect of high-frequency stimulation on synaptic transmission and intrinsic excitability at the mossy fiber-granule cell relay of the cerebellum. High-frequency stimulation, in addition to increasing synaptic conductance (D'Angelo et al., 1999), increased granule cell input resistance and decreased spike threshold. These changes depended on postsynaptic depolarization and NMDA receptor activation and were prevented by inhibitory synaptic activity. Potentiation of intrinsic excitability was induced by relatively weaker inputs than potentiation of synaptic efficacy, whereas with stronger inputs the two aspect of potentiation combined to enhance EPSPs and spike generation. Potentiation of intrinsic excitability may extend the computational capability of the cerebellar mossy fiber-granule cell relay.
Figures
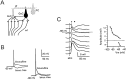
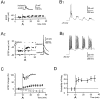
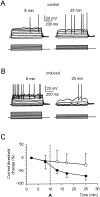
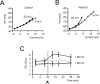
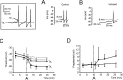
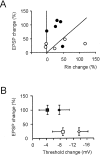
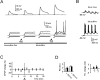
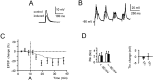
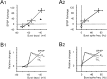
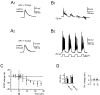
Similar articles
-
Evidence for NMDA and mGlu receptor-dependent long-term potentiation of mossy fiber-granule cell transmission in rat cerebellum.J Neurophysiol. 1999 Jan;81(1):277-87. doi: 10.1152/jn.1999.81.1.277. J Neurophysiol. 1999. PMID: 9914288
-
LTP regulates burst initiation and frequency at mossy fiber-granule cell synapses of rat cerebellum: experimental observations and theoretical predictions.J Neurophysiol. 2006 Feb;95(2):686-99. doi: 10.1152/jn.00696.2005. Epub 2005 Oct 5. J Neurophysiol. 2006. PMID: 16207782
-
Long-Term Potentiation at the Mossy Fiber-Granule Cell Relay Invokes Postsynaptic Second-Messenger Regulation of Kv4 Channels.J Neurosci. 2016 Nov 2;36(44):11196-11207. doi: 10.1523/JNEUROSCI.2051-16.2016. J Neurosci. 2016. PMID: 27807163 Free PMC article.
-
Long-term potentiation of synaptic transmission at the mossy fiber-granule cell relay of cerebellum.Prog Brain Res. 2005;148:69-80. doi: 10.1016/S0079-6123(04)48007-8. Prog Brain Res. 2005. PMID: 15661182 Review.
-
A peculiar form of potentiation in mossy fiber synapses.Epilepsy Res Suppl. 1992;7:151-7. Epilepsy Res Suppl. 1992. PMID: 1334660 Review.
Cited by
-
Distributed synergistic plasticity and cerebellar learning.Nat Rev Neurosci. 2012 Sep;13(9):619-35. doi: 10.1038/nrn3312. Epub 2012 Aug 16. Nat Rev Neurosci. 2012. PMID: 22895474 Review.
-
Integrated plasticity at inhibitory and excitatory synapses in the cerebellar circuit.Front Cell Neurosci. 2015 May 5;9:169. doi: 10.3389/fncel.2015.00169. eCollection 2015. Front Cell Neurosci. 2015. PMID: 25999817 Free PMC article. Review.
-
Intrinsic plasticity complements long-term potentiation in parallel fiber input gain control in cerebellar Purkinje cells.J Neurosci. 2010 Oct 13;30(41):13630-43. doi: 10.1523/JNEUROSCI.3226-10.2010. J Neurosci. 2010. PMID: 20943904 Free PMC article.
-
Metabotropic glutamate receptor subtype 1 regulates sodium currents in rat neocortical pyramidal neurons.J Physiol. 2006 Nov 15;577(Pt 1):141-54. doi: 10.1113/jphysiol.2006.118026. Epub 2006 Aug 24. J Physiol. 2006. PMID: 16931548 Free PMC article.
-
Distributed cerebellar plasticity implements adaptable gain control in a manipulation task: a closed-loop robotic simulation.Front Neural Circuits. 2013 Oct 9;7:159. doi: 10.3389/fncir.2013.00159. eCollection 2013. Front Neural Circuits. 2013. PMID: 24130518 Free PMC article.
References
-
- Aizenmann C, Linden DJ. Rapid, synaptically driven increases in the intrinsic excitability of cerebellar nuclear neurons. Nat Neurosci. 2000;3:109–111. - PubMed
-
- Aizenmann C, Manis PB, Linden DJ. Polarity of long-term synaptic changes is related to postsynaptic spike firing at a cerebellar inhibitory synapse. Neuron. 1998;21:827–835. - PubMed
-
- Arbib MA, Erdi P, Szentagothai J. Neural organization: structure, function, and dynamics. MIT; London: 1998. Cerebellum. pp. 261–302.
Publication types
MeSH terms
Substances
LinkOut - more resources
Full Text Sources