Mechanism for specificity by HMG-1 in enhanceosome assembly
- PMID: 10825199
- PMCID: PMC85803
- DOI: 10.1128/MCB.20.12.4359-4370.2000
Mechanism for specificity by HMG-1 in enhanceosome assembly
Abstract
Assembly of enhanceosomes requires architectural proteins to facilitate the DNA conformational changes accompanying cooperative binding of activators to a regulatory sequence. The architectural protein HMG-1 has been proposed to bind DNA in a sequence-independent manner, yet, paradoxically, it facilitates specific DNA binding reactions in vitro. To investigate the mechanism of specificity we explored the effect of HMG-1 on binding of the Epstein-Barr virus activator ZEBRA to a natural responsive promoter in vitro. DNase I footprinting, mutagenesis, and electrophoretic mobility shift assay reveal that HMG-1 binds cooperatively with ZEBRA to a specific DNA sequence between two adjacent ZEBRA recognition sites. This binding requires a strict alignment between two adjacent ZEBRA sites and both HMG boxes of HMG-1. Our study provides the first demonstration of sequence-dependent binding by a nonspecific HMG-box protein. We hypothesize how a ubiquitous, nonspecific architectural protein can function in a specific context through the use of rudimentary sequence recognition coupled with cooperativity. The observation that an abundant architectural protein can bind DNA cooperatively and specifically has implications towards understanding HMG-1's role in mediating DNA transactions in a variety of enzymological systems.
Figures
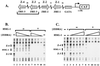
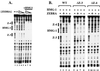
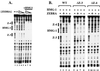
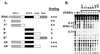
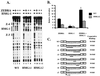
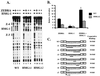
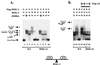
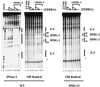
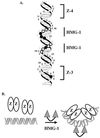
Similar articles
-
HMG domain proteins: architectural elements in the assembly of nucleoprotein structures.Trends Genet. 1994 Mar;10(3):94-100. doi: 10.1016/0168-9525(94)90232-1. Trends Genet. 1994. PMID: 8178371 Review.
-
A role of basic residues and the putative intercalating phenylalanine of the HMG-1 box B in DNA supercoiling and binding to four-way DNA junctions.J Biol Chem. 2000 Nov 17;275(46):35699-707. doi: 10.1074/jbc.M007167200. J Biol Chem. 2000. PMID: 10962007
-
The architectural transcription factor high mobility group I(Y) participates in photoreceptor-specific gene expression.J Neurosci. 2000 Oct 1;20(19):7317-24. doi: 10.1523/JNEUROSCI.20-19-07317.2000. J Neurosci. 2000. PMID: 11007889 Free PMC article.
-
The DNA architectural protein HMGB1 displays two distinct modes of action that promote enhanceosome assembly.Mol Cell Biol. 2002 Jun;22(12):4390-401. doi: 10.1128/MCB.22.12.4390-4401.2002. Mol Cell Biol. 2002. PMID: 12024049 Free PMC article.
-
HMG1 and 2: architectural DNA-binding proteins.Biochem Soc Trans. 2001 Aug;29(Pt 4):395-401. doi: 10.1042/bst0290395. Biochem Soc Trans. 2001. PMID: 11497996 Review.
Cited by
-
High-mobility group box 1: an amplifier of stem and progenitor cell activity after stroke.Acta Neurochir Suppl. 2013;118:31-8. doi: 10.1007/978-3-7091-1434-6_5. Acta Neurochir Suppl. 2013. PMID: 23564100 Free PMC article. Review.
-
Determinants of HMGB proteins required to promote RAG1/2-recombination signal sequence complex assembly and catalysis during V(D)J recombination.Mol Cell Biol. 2005 Jun;25(11):4413-25. doi: 10.1128/MCB.25.11.4413-4425.2005. Mol Cell Biol. 2005. PMID: 15899848 Free PMC article.
-
Both high mobility group (HMG)-boxes and the acidic tail of HMGB1 regulate recombination-activating gene (RAG)-mediated recombination signal synapsis and cleavage in vitro.J Biol Chem. 2005 Sep 2;280(35):31314-24. doi: 10.1074/jbc.M503063200. Epub 2005 Jul 1. J Biol Chem. 2005. PMID: 15994314 Free PMC article.
-
Identification of a TAL1 target gene reveals a positive role for the LIM domain-binding protein Ldb1 in erythroid gene expression and differentiation.Mol Cell Biol. 2003 Nov;23(21):7585-99. doi: 10.1128/MCB.23.21.7585-7599.2003. Mol Cell Biol. 2003. PMID: 14560005 Free PMC article.
-
Nucleosome dynamics: HMGB1 relaxes canonical nucleosome structure to facilitate estrogen receptor binding.Nucleic Acids Res. 2012 Nov 1;40(20):10161-71. doi: 10.1093/nar/gks815. Epub 2012 Aug 31. Nucleic Acids Res. 2012. PMID: 22941653 Free PMC article.
References
-
- Boonyaratanakornkit V, Melvin V, Prendergast P, Altmann M, Ronfani L, Bianchi M E, Taraseviciene L, Nordeen S K, Allegretto E A, Edwards D P. High-mobility group chromatin proteins 1 and 2 functionally interact with steroid hormone receptors to enhance their DNA binding in vitro and transcriptional activity in mammalian cells. Mol Cell Biol. 1998;18:4471–4487. - PMC - PubMed
-
- Bustin M, Reeves R. High-mobility-group chromosomal proteins: architectural components that facilitate chromatin function. Prog Nucleic Acid Res Mol Biol. 1996;54:35–100. - PubMed
Publication types
MeSH terms
Substances
Grants and funding
LinkOut - more resources
Full Text Sources