Microtubule interactions with the cell cortex causing nuclear movements in Saccharomyces cerevisiae
- PMID: 10811827
- PMCID: PMC2174570
- DOI: 10.1083/jcb.149.4.863
Microtubule interactions with the cell cortex causing nuclear movements in Saccharomyces cerevisiae
Abstract
During mitosis in budding yeast the nucleus first moves to the mother-bud neck and then into the neck. Both movements depend on interactions of cytoplasmic microtubules with the cortex. We investigated the mechanism of these movements in living cells using video analysis of GFP-labeled microtubules in wild-type cells and in EB1 and Arp1 mutants, which are defective in the first and second steps, respectively. We found that nuclear movement to the neck is largely mediated by the capture of microtubule ends at one cortical region at the incipient bud site or bud tip, followed by microtubule depolymerization. Efficient microtubule interactions with the capture site require that microtubules be sufficiently long and dynamic to probe the cortex. In contrast, spindle movement into the neck is mediated by microtubule sliding along the bud cortex, which requires dynein and dynactin. Free microtubules can also slide along the cortex of both bud and mother. Capture/shrinkage of microtubule ends also contributes to nuclear movement into the neck and can serve as a backup mechanism to move the nucleus into the neck when microtubule sliding is impaired. Conversely, microtubule sliding can move the nucleus into the neck even when capture/shrinkage is impaired.
Figures
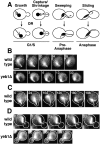
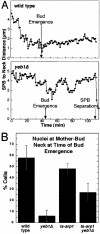
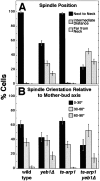
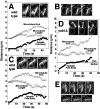
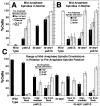
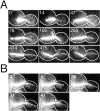
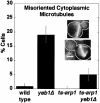
Similar articles
-
The role of the lissencephaly protein Pac1 during nuclear migration in budding yeast.J Cell Biol. 2003 Feb 3;160(3):355-64. doi: 10.1083/jcb.200209022. J Cell Biol. 2003. PMID: 12566428 Free PMC article.
-
The cortical protein Num1p is essential for dynein-dependent interactions of microtubules with the cortex.J Cell Biol. 2000 Dec 11;151(6):1337-44. doi: 10.1083/jcb.151.6.1337. J Cell Biol. 2000. PMID: 11121446 Free PMC article.
-
Dynamic positioning of mitotic spindles in yeast: role of microtubule motors and cortical determinants.Mol Biol Cell. 2000 Nov;11(11):3949-61. doi: 10.1091/mbc.11.11.3949. Mol Biol Cell. 2000. PMID: 11071919 Free PMC article.
-
Mitotic motors in Saccharomyces cerevisiae.Biochim Biophys Acta. 2000 Mar 17;1496(1):99-116. doi: 10.1016/s0167-4889(00)00012-4. Biochim Biophys Acta. 2000. PMID: 10722880 Review.
-
[Dynein and dynactin as organizers of the system of cell microtubules].Ontogenez. 2006 Sep-Oct;37(5):323-39. Ontogenez. 2006. PMID: 17066975 Review. Russian.
Cited by
-
Stopped in its tracks: negative regulation of the dynein motor by the yeast protein She1.Bioessays. 2013 Aug;35(8):677-82. doi: 10.1002/bies.201300016. Epub 2013 May 13. Bioessays. 2013. PMID: 23666903 Free PMC article.
-
The Bud14p-Glc7p complex functions as a cortical regulator of dynein in budding yeast.EMBO J. 2005 Sep 7;24(17):3000-11. doi: 10.1038/sj.emboj.7600783. Epub 2005 Aug 18. EMBO J. 2005. PMID: 16107882 Free PMC article.
-
PAR-dependent and geometry-dependent mechanisms of spindle positioning.J Cell Biol. 2003 Mar 17;160(6):845-55. doi: 10.1083/jcb.200209079. J Cell Biol. 2003. PMID: 12642612 Free PMC article.
-
Microtubule capture by the cleavage apparatus is required for proper spindle positioning in yeast.Genes Dev. 2002 Jul 1;16(13):1627-39. doi: 10.1101/gad.222602. Genes Dev. 2002. PMID: 12101122 Free PMC article.
-
Cytoplasmic dynein's mitotic spindle pole localization requires a functional anaphase-promoting complex, gamma-tubulin, and NUDF/LIS1 in Aspergillus nidulans.Mol Biol Cell. 2005 Aug;16(8):3591-605. doi: 10.1091/mbc.e04-12-1071. Epub 2005 Jun 1. Mol Biol Cell. 2005. PMID: 15930134 Free PMC article.
References
Publication types
MeSH terms
Substances
Grants and funding
LinkOut - more resources
Full Text Sources
Other Literature Sources
Molecular Biology Databases