Mutational analysis of mammalian translation initiation factor 5 (eIF5): role of interaction between the beta subunit of eIF2 and eIF5 in eIF5 function in vitro and in vivo
- PMID: 10805737
- PMCID: PMC85746
- DOI: 10.1128/MCB.20.11.3942-3950.2000
Mutational analysis of mammalian translation initiation factor 5 (eIF5): role of interaction between the beta subunit of eIF2 and eIF5 in eIF5 function in vitro and in vivo
Abstract
Eukaryotic translation initiation factor 5 (eIF5) interacts with the 40S initiation complex (40S-eIF3-AUG-Met-tRNA(f)-eIF2-GTP) to promote the hydrolysis of ribosome-bound GTP. eIF5 also forms a complex with eIF2 by interacting with the beta subunit of eIF2. In this work, we have used a mutational approach to investigate the importance of eIF5-eIF2beta interaction in eIF5 function. Binding analyses with recombinant rat eIF5 deletion mutants identified the C terminus of eIF5 as the eIF2beta-binding region. Alanine substitution mutagenesis at sites within this region defined several conserved glutamic acid residues in a bipartite motif as critical for eIF5 function. The E346A,E347A and E384A,E385A double-point mutations each caused a severe defect in the binding of eIF5 to eIF2beta but not to eIF3-Nip1p, while a eIF5 hexamutant (E345A,E346A, E347A,E384A,E385A,E386A) showed negligible binding to eIF2beta. These mutants were also severely defective in eIF5-dependent GTP hydrolysis, in 80S initiation complex formation, and in the ability to stimulate translation of mRNAs in an eIF5-dependent yeast cell-free translation system. Furthermore, unlike wild-type rat eIF5, which can functionally substitute for yeast eIF5 in complementing in vivo a genetic disruption of the chromosomal copy of the TIF5 gene, the eIF5 double-point mutants allowed only slow growth of this DeltaTIF5 yeast strain, while the eIF5 hexamutant was unable to support cell growth and viability of this strain. These findings suggest that eIF5-eIF2beta interaction plays an essential role in eIF5 function in eukaryotic cells.
Figures
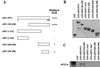
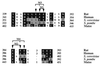
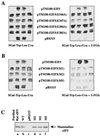
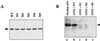
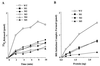
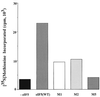
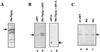
Similar articles
-
Multiple roles for the C-terminal domain of eIF5 in translation initiation complex assembly and GTPase activation.EMBO J. 2001 May 1;20(9):2326-37. doi: 10.1093/emboj/20.9.2326. EMBO J. 2001. PMID: 11331597 Free PMC article.
-
Isolation and functional characterization of a temperature-sensitive mutant of the yeast Saccharomyces cerevisiae in translation initiation factor eIF5: an eIF5-dependent cell-free translation system.Gene. 2000 Feb 22;244(1-2):109-18. doi: 10.1016/s0378-1119(99)00570-3. Gene. 2000. PMID: 10689193
-
A multifactor complex of eukaryotic initiation factors, eIF1, eIF2, eIF3, eIF5, and initiator tRNA(Met) is an important translation initiation intermediate in vivo.Genes Dev. 2000 Oct 1;14(19):2534-46. doi: 10.1101/gad.831800. Genes Dev. 2000. PMID: 11018020 Free PMC article.
-
Functional significance and mechanism of eIF5-promoted GTP hydrolysis in eukaryotic translation initiation.Prog Nucleic Acid Res Mol Biol. 2001;70:207-31. doi: 10.1016/s0079-6603(01)70018-9. Prog Nucleic Acid Res Mol Biol. 2001. PMID: 11642363 Review.
-
A multifactor complex of eIF1, eIF2, eIF3, eIF5, and tRNA(i)Met promotes initiation complex assembly and couples GTP hydrolysis to AUG recognition.Cold Spring Harb Symp Quant Biol. 2001;66:403-15. doi: 10.1101/sqb.2001.66.403. Cold Spring Harb Symp Quant Biol. 2001. PMID: 12762043 Review. No abstract available.
Cited by
-
Multiple roles for the C-terminal domain of eIF5 in translation initiation complex assembly and GTPase activation.EMBO J. 2001 May 1;20(9):2326-37. doi: 10.1093/emboj/20.9.2326. EMBO J. 2001. PMID: 11331597 Free PMC article.
-
Deletion of eIF2β lysine stretches creates a dominant negative that affects the translation and proliferation in human cell line: A tool for arresting the cell growth.Cancer Biol Ther. 2017 Aug 3;18(8):560-570. doi: 10.1080/15384047.2017.1345383. Epub 2017 Jul 10. Cancer Biol Ther. 2017. PMID: 28692326 Free PMC article.
-
Regulation of GTP hydrolysis prior to ribosomal AUG selection during eukaryotic translation initiation.EMBO J. 2005 Nov 2;24(21):3737-46. doi: 10.1038/sj.emboj.7600844. Epub 2005 Oct 13. EMBO J. 2005. PMID: 16222335 Free PMC article.
-
Assessing the components of the eIF3 complex and their phosphorylation status.J Proteome Res. 2011 Apr 1;10(4):1481-94. doi: 10.1021/pr100877m. Epub 2011 Mar 2. J Proteome Res. 2011. PMID: 21280672 Free PMC article.
-
The archaeal eIF2 homologue: functional properties of an ancient translation initiation factor.Nucleic Acids Res. 2005 Mar 23;33(6):1804-12. doi: 10.1093/nar/gki321. Print 2005. Nucleic Acids Res. 2005. PMID: 15788752 Free PMC article.
References
-
- Bourne H R, Sanders D A, McCormick F. The GTPase superfamily: conserved structure and molecular mechanism. Nature. 1991;349:117–127. - PubMed
-
- Chakrabarti A, Maitra U. Function of eukaryotic initiation factor 5 in the formation of an 80S ribosomal polypeptide chain initiation complex. J Biol Chem. 1991;266:14039–14045. - PubMed
-
- Chakravarti D, Maiti T, Maitra U. Isolation and immunochemical characterization of eukaryotic translation initiation factor 5 from Saccharomyces cerevisiae. J Biol Chem. 1993;268:5754–5762. - PubMed
Publication types
MeSH terms
Substances
Grants and funding
LinkOut - more resources
Full Text Sources
Other Literature Sources
Molecular Biology Databases
Miscellaneous