Mammalian spindle orientation and position respond to changes in cell shape in a dynein-dependent fashion
- PMID: 10793150
- PMCID: PMC14882
- DOI: 10.1091/mbc.11.5.1765
Mammalian spindle orientation and position respond to changes in cell shape in a dynein-dependent fashion
Abstract
In animal cells, positioning of the mitotic spindle is crucial for defining the plane of cytokinesis and the size ratio of daughter cells. We have characterized this phenomenon in a rat epithelial cell line using microscopy, micromanipulation, and microinjection. Unmanipulated cells position the mitotic spindle near their geometric center, with the spindle axis lying roughly parallel to the long axis of the cell. Spindles that were initially misoriented underwent directed rotation and caused a delay in anaphase onset. To gain further insight into this process, we gently deformed cells with a blunted glass needle to change the spatial relationship between the cortex and spindle. This manipulation induced spindle movement or rotation in metaphase and/or anaphase, until the spindle reached a proper position relative to the deformed shape. Spindle positioning was inhibited by either treatment with low doses of nocodazole or microinjection of antibodies against dynein, apparently due to the disruption of the organization of dynein and/or astral microtubules. Our results suggest that mitotic cells continuously monitor and maintain the position of the spindle relative to the cortex. This process is likely driven by interactions among astral microtubules, the motor protein dynein, and the cell cortex and may constitute part of a mitotic checkpoint mechanism.
Figures
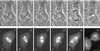
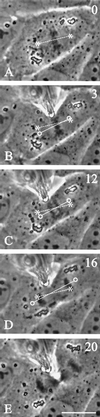
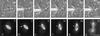
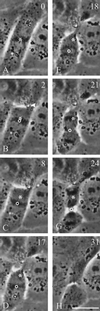
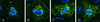
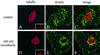
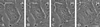
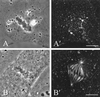
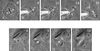
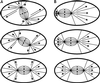
Similar articles
-
Dynamic positioning of mitotic spindles in yeast: role of microtubule motors and cortical determinants.Mol Biol Cell. 2000 Nov;11(11):3949-61. doi: 10.1091/mbc.11.11.3949. Mol Biol Cell. 2000. PMID: 11071919 Free PMC article.
-
RanGTP and CLASP1 cooperate to position the mitotic spindle.Mol Biol Cell. 2013 Aug;24(16):2506-14. doi: 10.1091/mbc.E13-03-0150. Epub 2013 Jun 19. Mol Biol Cell. 2013. PMID: 23783028 Free PMC article.
-
Astral microtubules control redistribution of dynein at the cell cortex to facilitate spindle positioning.Cell Cycle. 2014;13(7):1162-70. doi: 10.4161/cc.28031. Epub 2014 Feb 10. Cell Cycle. 2014. PMID: 24553118 Free PMC article.
-
Mechanisms of daughter cell-size control during cell division.Trends Cell Biol. 2015 May;25(5):286-95. doi: 10.1016/j.tcb.2014.12.003. Epub 2014 Dec 26. Trends Cell Biol. 2015. PMID: 25548067 Review.
-
Physical Limits on the Precision of Mitotic Spindle Positioning by Microtubule Pushing forces: Mechanics of mitotic spindle positioning.Bioessays. 2017 Nov;39(11):10.1002/bies.201700122. doi: 10.1002/bies.201700122. Epub 2017 Sep 28. Bioessays. 2017. PMID: 28960439 Free PMC article. Review.
Cited by
-
Effect of Cell Shape and Dimensionality on Spindle Orientation and Mitotic Timing.PLoS One. 2013 Jun 18;8(6):e66918. doi: 10.1371/journal.pone.0066918. Print 2013. PLoS One. 2013. PMID: 23825020 Free PMC article.
-
Micro- and nanoscale engineering of cell signaling.Annu Rev Biomed Eng. 2013;15:305-26. doi: 10.1146/annurev-bioeng-071811-150050. Annu Rev Biomed Eng. 2013. PMID: 23862677 Free PMC article. Review.
-
Dynein-mediated pulling forces drive rapid mitotic spindle elongation in Ustilago maydis.EMBO J. 2006 Oct 18;25(20):4897-908. doi: 10.1038/sj.emboj.7601354. Epub 2006 Oct 5. EMBO J. 2006. PMID: 17024185 Free PMC article.
-
Phospho-regulated interaction between kinesin-6 Klp9p and microtubule bundler Ase1p promotes spindle elongation.Dev Cell. 2009 Aug;17(2):257-67. doi: 10.1016/j.devcel.2009.06.012. Dev Cell. 2009. PMID: 19686686 Free PMC article.
-
The transforming parasite Theileria co-opts host cell mitotic and central spindles to persist in continuously dividing cells.PLoS Biol. 2010 Sep 28;8(9):e1000499. doi: 10.1371/journal.pbio.1000499. PLoS Biol. 2010. PMID: 20927361 Free PMC article.
References
-
- Busson S, Dujardin D, Moreau A, Dompierre J, DeMey JR. Dynein and dynactin are localized to astral microtubules and at cortical sites in mitotic epithelial cells. Curr Biol. 1998;8:541–544. - PubMed
Publication types
MeSH terms
Substances
Grants and funding
LinkOut - more resources
Full Text Sources
Miscellaneous