Directional transneuronal infection by pseudorabies virus is dependent on an acidic internalization motif in the Us9 cytoplasmic tail
- PMID: 10775591
- PMCID: PMC111975
- DOI: 10.1128/jvi.74.10.4549-4561.2000
Directional transneuronal infection by pseudorabies virus is dependent on an acidic internalization motif in the Us9 cytoplasmic tail
Abstract
The Us9 gene is conserved among most alphaherpesviruses. In pseudorabies virus (PRV), the Us9 protein is a 98-amino-acid, type II membrane protein found in the virion envelope. It localizes to the trans-Golgi network (TGN) region in infected and transfected cells and is maintained in this compartment by endocytosis from the plasma membrane. Viruses with Us9 deleted have no observable defects in tissue culture yet have reduced virulence and restricted spread to retinorecipient neurons in the rodent brain. In this report, we demonstrate that Us9-promoted transneuronal spread in vivo is dependent on a conserved acidic motif previously shown to be essential for the maintenance of Us9 in the TGN region and recycling from the plasma membrane. Mutant viruses with the acidic motif deleted have an anterograde spread defect indistinguishable from that of Us9 null viruses. Transneuronal spread, however, is not dependent on a dileucine endocytosis motif in the Us9 cytoplasmic tail. Through alanine scanning mutagenesis of the acidic motif, we have identified two conserved tyrosine residues that are essential for Us9-mediated spread as well as two serine residues, comprising putative consensus casein kinase II sites, that modulate the rate of PRV transneuronal spread in vivo.
Figures
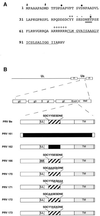
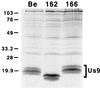
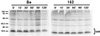
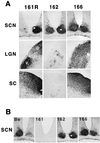
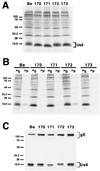
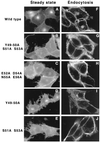
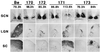
Similar articles
-
Role of pseudorabies virus Us9, a type II membrane protein, in infection of tissue culture cells and the rat nervous system.J Virol. 2000 Jan;74(2):834-45. doi: 10.1128/jvi.74.2.834-845.2000. J Virol. 2000. PMID: 10623746 Free PMC article.
-
Role of Us9 phosphorylation in axonal sorting and anterograde transport of pseudorabies virus.PLoS One. 2013;8(3):e58776. doi: 10.1371/journal.pone.0058776. Epub 2013 Mar 19. PLoS One. 2013. PMID: 23527020 Free PMC article.
-
Intracellular trafficking and localization of the pseudorabies virus Us9 type II envelope protein to host and viral membranes.J Virol. 1999 May;73(5):4372-84. doi: 10.1128/JVI.73.5.4372-4384.1999. J Virol. 1999. PMID: 10196335 Free PMC article.
-
The role of virion membrane protein endocytosis in the herpesvirus life cycle.J Clin Virol. 2000 Aug;17(2):69-82. doi: 10.1016/s1386-6532(00)00084-6. J Clin Virol. 2000. PMID: 10942087 Review.
-
Practical considerations for the use of pseudorabies virus in transneuronal studies of neural circuitry.Neurosci Biobehav Rev. 1998 Oct;22(6):685-94. doi: 10.1016/s0149-7634(98)00007-4. Neurosci Biobehav Rev. 1998. PMID: 9809304 Review.
Cited by
-
Comparison of the pseudorabies virus Us9 protein with homologs from other veterinary and human alphaherpesviruses.J Virol. 2009 Jul;83(14):6978-86. doi: 10.1128/JVI.00598-09. Epub 2009 May 6. J Virol. 2009. PMID: 19420087 Free PMC article.
-
Herpes simplex virus membrane proteins gE/gI and US9 act cooperatively to promote transport of capsids and glycoproteins from neuron cell bodies into initial axon segments.J Virol. 2013 Jan;87(1):403-14. doi: 10.1128/JVI.02465-12. Epub 2012 Oct 17. J Virol. 2013. PMID: 23077321 Free PMC article.
-
Neuron-to-cell spread of pseudorabies virus in a compartmented neuronal culture system.J Virol. 2005 Sep;79(17):10875-89. doi: 10.1128/JVI.79.17.10875-10889.2005. J Virol. 2005. PMID: 16103140 Free PMC article.
-
Herpes simplex virus tegument protein US11 interacts with conventional kinesin heavy chain.J Virol. 2002 Apr;76(7):3282-91. doi: 10.1128/jvi.76.7.3282-3291.2002. J Virol. 2002. PMID: 11884553 Free PMC article.
-
Fusion of enhanced green fluorescent protein to the pseudorabies virus axonal sorting protein Us9 blocks anterograde spread of infection in mammalian neurons.J Virol. 2008 Oct;82(20):10308-11. doi: 10.1128/JVI.01204-08. Epub 2008 Aug 6. J Virol. 2008. PMID: 18684822 Free PMC article.
References
-
- Alconada A, Bauer U, Baudoux L, Piette J, Hoflack B. Intracellular transport of the glycoproteins gE and gI of the varicella-zoster virus. J Biol Chem. 1998;273:13430–13436. - PubMed
-
- Becker C H. Zur primaren Schadingung vegetativer Ganglien nach Infektion mit dem Herpes suis Virus bei verschiedenen Tierarten. Experientia. 1967;23:209. - PubMed
-
- Ben-Porat T, Kaplan A S. Molecular biology of pseudorabies virus. In: Roizman B, editor. The herpesviruses. New York, N.Y: Plenum Publishing Corp.; 1985. pp. 105–173.
Publication types
MeSH terms
Substances
Grants and funding
LinkOut - more resources
Full Text Sources
Other Literature Sources
Miscellaneous