Functional coupling of Ca(2+) channels to ryanodine receptors at presynaptic terminals. Amplification of exocytosis and plasticity
- PMID: 10736317
- PMCID: PMC2233761
- DOI: 10.1085/jgp.115.4.519
Functional coupling of Ca(2+) channels to ryanodine receptors at presynaptic terminals. Amplification of exocytosis and plasticity
Abstract
Ca(2+)-induced Ca(2+) release (CICR) enhances a variety of cellular Ca(2+) signaling and functions. How CICR affects impulse-evoked transmitter release is unknown. At frog motor nerve terminals, repetitive Ca(2+) entries slowly prime and subsequently activate the mechanism of CICR via ryanodine receptors and asynchronous exocytosis of transmitters. Further Ca(2+) entry inactivates the CICR mechanism and the absence of Ca(2+) entry for >1 min results in its slow depriming. We now report here that the activation of this unique CICR markedly enhances impulse-evoked exocytosis of transmitter. The conditioning nerve stimulation (10-20 Hz, 2-10 min) that primes the CICR mechanism produced the marked enhancement of the amplitude and quantal content of end-plate potentials (EPPs) that decayed double exponentially with time constants of 1.85 and 10 min. The enhancement was blocked by inhibitors of ryanodine receptors and was accompanied by a slight prolongation of the peak times of EPP and the end-plate currents estimated from deconvolution of EPP. The conditioning nerve stimulation also enhanced single impulse- and tetanus-induced rises in intracellular Ca(2+) in the terminals with little change in time course. There was no change in the rate of growth of the amplitudes of EPPs in a short train after the conditioning stimulation. On the other hand, the augmentation and potentiation of EPP were enhanced, and then decreased in parallel with changes in intraterminal Ca(2+) during repetition of tetani. The results suggest that ryanodine receptors exist close to voltage-gated Ca(2+) channels in the presynaptic terminals and amplify the impulse-evoked exocytosis and its plasticity via CICR after Ca(2+)-dependent priming.
Figures
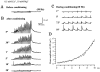
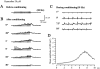
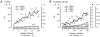
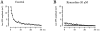
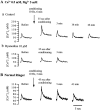
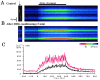
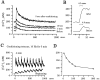
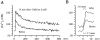
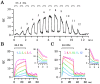
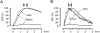
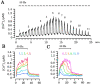
Similar articles
-
Type-3 ryanodine receptor involved in Ca2+-induced Ca2+ release and transmitter exocytosis at frog motor nerve terminals.Cell Calcium. 2005 Dec;38(6):557-67. doi: 10.1016/j.ceca.2005.07.008. Epub 2005 Sep 12. Cell Calcium. 2005. PMID: 16157373
-
A Ca2+-induced Ca2+ release mechanism involved in asynchronous exocytosis at frog motor nerve terminals.J Gen Physiol. 1998 Nov;112(5):593-609. doi: 10.1085/jgp.112.5.593. J Gen Physiol. 1998. PMID: 9806968 Free PMC article.
-
Calcium dependence of the priming, activation and inactivation of ryanodine receptors in frog motor nerve terminals.Eur J Neurosci. 2010 Sep;32(6):948-62. doi: 10.1111/j.1460-9568.2010.07381.x. Epub 2010 Aug 26. Eur J Neurosci. 2010. PMID: 20796022
-
Calcium channel regulation and presynaptic plasticity.Neuron. 2008 Sep 25;59(6):882-901. doi: 10.1016/j.neuron.2008.09.005. Neuron. 2008. PMID: 18817729 Review.
-
Presynaptic quantal plasticity: Katz's original hypothesis revisited.Synapse. 2003 Mar;47(3):184-99. doi: 10.1002/syn.10161. Synapse. 2003. PMID: 12494401 Review.
Cited by
-
cAMP-regulated guanine nucleotide exchange factor II (Epac2) mediates Ca2+-induced Ca2+ release in INS-1 pancreatic beta-cells.J Physiol. 2001 Oct 15;536(Pt 2):375-85. doi: 10.1111/j.1469-7793.2001.0375c.xd. J Physiol. 2001. PMID: 11600673 Free PMC article.
-
Calcium release from presynaptic ryanodine-sensitive stores is required for long-term depression at hippocampal CA3-CA3 pyramidal neuron synapses.J Neurosci. 2004 Oct 27;24(43):9612-22. doi: 10.1523/JNEUROSCI.5583-03.2004. J Neurosci. 2004. PMID: 15509748 Free PMC article.
-
Ryanodine receptors in peritoneal mast cells: possible role in the modulation of exocytotic activity.Pflugers Arch. 2004 Jan;447(4):377-86. doi: 10.1007/s00424-003-1196-7. Epub 2003 Nov 21. Pflugers Arch. 2004. PMID: 14634821
-
The Ever-Growing Puzzle of Asynchronous Release.Front Cell Neurosci. 2019 Feb 12;13:28. doi: 10.3389/fncel.2019.00028. eCollection 2019. Front Cell Neurosci. 2019. PMID: 30809127 Free PMC article. Review.
-
Bioluminescence imaging of mitochondrial Ca2+ dynamics in soma and neurites of individual adult mouse sympathetic neurons.J Physiol. 2007 Apr 15;580(Pt. 2):385-95. doi: 10.1113/jphysiol.2006.126524. Epub 2007 Jan 18. J Physiol. 2007. PMID: 17234693 Free PMC article.
References
Publication types
MeSH terms
Substances
LinkOut - more resources
Full Text Sources
Miscellaneous