DNA interstrand cross-links induce futile repair synthesis in mammalian cell extracts
- PMID: 10713168
- PMCID: PMC85433
- DOI: 10.1128/MCB.20.7.2446-2454.2000
DNA interstrand cross-links induce futile repair synthesis in mammalian cell extracts
Abstract
DNA interstrand cross-links are induced by many carcinogens and anticancer drugs. It was previously shown that mammalian DNA excision repair nuclease makes dual incisions 5' to the cross-linked base of a psoralen cross-link, generating a gap of 22 to 28 nucleotides adjacent to the cross-link. We wished to find the fates of the gap and the cross-link in this complex structure under conditions conducive to repair synthesis, using cell extracts from wild-type and cross-linker-sensitive mutant cell lines. We found that the extracts from both types of strains filled in the gap but were severely defective in ligating the resulting nick and incapable of removing the cross-link. The net result was a futile damage-induced DNA synthesis which converted a gap into a nick without removing the damage. In addition, in this study, we showed that the structure-specific endonuclease, the XPF-ERCC1 heterodimer, acted as a 3'-to-5' exonuclease on cross-linked DNA in the presence of RPA. Collectively, these observations shed some light on the cellular processing of DNA cross-links and reveal that cross-links induce a futile DNA synthesis cycle that may constitute a signal for specific cellular responses to cross-linked DNA.
Figures
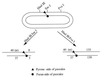
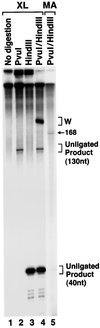
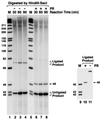
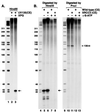
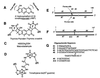
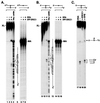
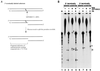
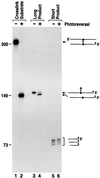
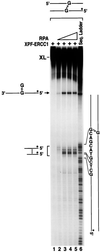
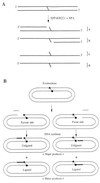
Similar articles
-
Interstrand cross-links induce DNA synthesis in damaged and undamaged plasmids in mammalian cell extracts.Mol Cell Biol. 1999 Aug;19(8):5619-30. doi: 10.1128/MCB.19.8.5619. Mol Cell Biol. 1999. PMID: 10409751 Free PMC article.
-
Repair of an interstrand DNA cross-link initiated by ERCC1-XPF repair/recombination nuclease.J Biol Chem. 2000 Aug 25;275(34):26632-6. doi: 10.1074/jbc.C000337200. J Biol Chem. 2000. PMID: 10882712
-
Processing of a psoralen DNA interstrand cross-link by XPF-ERCC1 complex in vitro.J Biol Chem. 2008 Jan 18;283(3):1275-1281. doi: 10.1074/jbc.M708072200. Epub 2007 Nov 15. J Biol Chem. 2008. PMID: 18006494
-
A role for the base excision repair enzyme NEIL3 in replication-dependent repair of interstrand DNA cross-links derived from psoralen and abasic sites.DNA Repair (Amst). 2017 Apr;52:1-11. doi: 10.1016/j.dnarep.2017.02.011. Epub 2017 Feb 20. DNA Repair (Amst). 2017. PMID: 28262582 Free PMC article. Review.
-
Interstrand crosslink repair: can XPF-ERCC1 be let off the hook?Trends Genet. 2008 Feb;24(2):70-6. doi: 10.1016/j.tig.2007.11.003. Epub 2008 Jan 14. Trends Genet. 2008. PMID: 18192062 Review.
Cited by
-
Formation and repair of interstrand cross-links in DNA.Chem Rev. 2006 Feb;106(2):277-301. doi: 10.1021/cr040478b. Chem Rev. 2006. PMID: 16464006 Free PMC article. Review. No abstract available.
-
Targeting Chromosomal Architectural HMGB Proteins Could Be the Next Frontier in Cancer Therapy.Cancer Res. 2020 Jun 1;80(11):2075-2082. doi: 10.1158/0008-5472.CAN-19-3066. Epub 2020 Mar 9. Cancer Res. 2020. PMID: 32152151 Free PMC article. Review.
-
Role of Base Excision Repair Pathway in the Processing of Complex DNA Damage Generated by Oxidative Stress and Anticancer Drugs.Front Cell Dev Biol. 2021 Jan 22;8:617884. doi: 10.3389/fcell.2020.617884. eCollection 2020. Front Cell Dev Biol. 2021. PMID: 33553154 Free PMC article. Review.
-
Role for DNA polymerase kappa in the processing of N2-N2-guanine interstrand cross-links.J Biol Chem. 2008 Jun 20;283(25):17075-82. doi: 10.1074/jbc.M801238200. Epub 2008 Apr 22. J Biol Chem. 2008. PMID: 18434313 Free PMC article.
-
Distortion-dependent unhooking of interstrand cross-links in mammalian cell extracts.Biochemistry. 2008 Sep 16;47(37):9920-30. doi: 10.1021/bi800925e. Epub 2008 Aug 15. Biochemistry. 2008. PMID: 18702509 Free PMC article.
References
-
- Bessho T, Sancar A, Thompson L H, Thelen M P. Reconstitution of human excision nuclease with recombinant XPF-ERCC1 complex. J Biol Chem. 1997;272:3833–3837. - PubMed
-
- Cimino G D, Gamper H B, Isaacs S T, Hearst J E. Psoralens as photoactive probes of nucleic acid structure and function: organic chemistry, photochemistry, and biochemistry. Annu Rev Biochem. 1985;54:1151–1193. - PubMed
Publication types
MeSH terms
Substances
Grants and funding
LinkOut - more resources
Full Text Sources
Miscellaneous