The first alpha helix of interleukin (IL)-2 folds as a homotetramer, acts as an agonist of the IL-2 receptor beta chain, and induces lymphokine-activated killer cells
- PMID: 10662798
- PMCID: PMC2195822
- DOI: 10.1084/jem.191.3.529
The first alpha helix of interleukin (IL)-2 folds as a homotetramer, acts as an agonist of the IL-2 receptor beta chain, and induces lymphokine-activated killer cells
Abstract
Interleukin (IL)-2 interacts with two types of functional receptors (IL-2Ralphabetagamma and IL-2Rbetagamma) and acts on a broad range of target cells involved in inflammatory reactions and immune responses. For the first time, we show that a chemically synthesized fragment of the IL-2 sequence can fold into a molecule mimicking the quaternary structure of a hemopoietin. Indeed, peptide p1-30 (containing amino acids 1-30, covering the entire alpha helix A of IL-2) spontaneously folds into an alpha-helical homotetramer and stimulates the growth of T cell lines expressing human IL-2Rbeta, whereas shorter versions of the peptide lack helical structure and are inactive. We also demonstrate that this neocytokine interacts with a previously undescribed dimeric form of IL-2Rbeta. In agreement with its binding to IL-2Rbeta, p1-30 activates Shc and p56(lck) but unlike IL-2, fails to activate Janus kinase (Jak)1, Jak3, and signal transducer and activator of transcription 5 (STAT5). Unexpectedly, we also show that p1-30 activates Tyk2, thus suggesting that IL-2Rbeta may bind to different Jaks depending on its oligomerization. At the cellular level, p1-30 induces lymphokine-activated killer (LAK) cells and preferentially activates CD8(low) lymphocytes and natural killer cells, which constitutively express IL-2Rbeta. A significant interferon gamma production is also detected after p1-30 stimulation. A mutant form of p1-30 (Asp20-->Lys), which is likely unable to induce vascular leak syndrome, remains capable of generating LAK cells, like the original p1-30 peptide. Altogether, our data suggest that p1-30 has therapeutic potential.
Figures
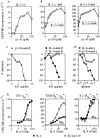
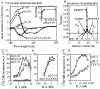
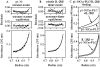
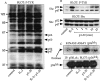
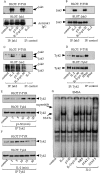
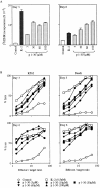
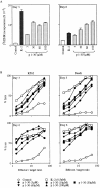
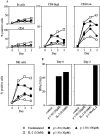
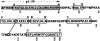
Similar articles
-
Characterization of an IL-2 mimetic with therapeutic potential.Cell Mol Biol (Noisy-le-grand). 2001 Jun;47(4):703-7. Cell Mol Biol (Noisy-le-grand). 2001. PMID: 11502078 Review.
-
IL-2R beta agonist P1-30 acts in synergy with IL-2, IL-4, IL-9, and IL-15: biological and molecular effects.J Immunol. 2000 Oct 15;165(8):4312-8. doi: 10.4049/jimmunol.165.8.4312. J Immunol. 2000. PMID: 11035066
-
Structural analysis and modeling of a synthetic interleukin-2 mimetic and its interleukin-2Rbeta2 receptor.J Biol Chem. 2003 Jun 20;278(25):22868-76. doi: 10.1074/jbc.M301757200. Epub 2003 Apr 3. J Biol Chem. 2003. PMID: 12676936
-
IL-2 induces conformational changes in its preassembled receptor core, which then migrates in lipid raft and binds to the cytoskeleton meshwork.J Mol Biol. 2010 Nov 12;403(5):671-92. doi: 10.1016/j.jmb.2010.08.056. Epub 2010 Sep 21. J Mol Biol. 2010. PMID: 20816854
-
IL-21: a novel IL-2-family lymphokine that modulates B, T, and natural killer cell responses.J Allergy Clin Immunol. 2003 Dec;112(6):1033-45. doi: 10.1016/j.jaci.2003.08.039. J Allergy Clin Immunol. 2003. PMID: 14657853 Review.
Cited by
-
Construction, purification, and characterization of a chimeric TH1 antagonist.BMC Biotechnol. 2006 May 22;6:25. doi: 10.1186/1472-6750-6-25. BMC Biotechnol. 2006. PMID: 16716222 Free PMC article.
-
In silico identification of opossum cytokine genes suggests the complexity of the marsupial immune system rivals that of eutherian mammals.Immunome Res. 2006 Nov 10;2:4. doi: 10.1186/1745-7580-2-4. Immunome Res. 2006. PMID: 17094811 Free PMC article.
-
Interleukin-7 compartmentalizes its receptor signaling complex to initiate CD4 T lymphocyte response.J Biol Chem. 2010 May 14;285(20):14898-14908. doi: 10.1074/jbc.M110.104232. Epub 2010 Feb 18. J Biol Chem. 2010. Retraction in: J Biol Chem. 2019 Jan 25;294(4):1436. doi: 10.1074/jbc.W118.007350 PMID: 20167604 Free PMC article. Retracted.
-
Characterisation and expression analysis of interleukin 2 (IL-2) and IL-21 homologues in the Japanese pufferfish, Fugu rubripes, following their discovery by synteny.Immunogenetics. 2005 Mar;56(12):909-23. doi: 10.1007/s00251-004-0741-7. Epub 2004 Dec 11. Immunogenetics. 2005. PMID: 15592926
References
-
- Taniguchi T., Matsui H., Fujita T., Takaoka C., Kashima N., Yoshimoto R., Hamuro J. Structure and expression of a cloned cDNA for human interleukin-2. Nature. 1983;302:305–310. - PubMed
-
- MacKay D. Unraveling the structure of IL-2reply. Science. 1992;257:410–413. - PubMed
-
- Smith K.A. Interleukin-2inception, impact and implications. Science. 1988;240:1169–1176. - PubMed
-
- Thèze J., Alzari P.M., Bertoglio J. Interleukin 2 and its receptorsrecent advances and new immunological functions. Immunol. Today. 1996;10:481–486. - PubMed
Publication types
MeSH terms
Substances
LinkOut - more resources
Full Text Sources
Other Literature Sources
Research Materials
Miscellaneous