RAD51 is required for the repair of plasmid double-stranded DNA gaps from either plasmid or chromosomal templates
- PMID: 10648605
- PMCID: PMC85244
- DOI: 10.1128/MCB.20.4.1194-1205.2000
RAD51 is required for the repair of plasmid double-stranded DNA gaps from either plasmid or chromosomal templates
Abstract
DNA double-strand breaks may be induced by endonucleases, ionizing radiation, chemical agents, and mechanical forces or by replication of single-stranded nicked chromosomes. Repair of double-strand breaks can occur by homologous recombination or by nonhomologous end joining. A system was developed to measure the efficiency of plasmid gap repair by homologous recombination using either chromosomal or plasmid templates. Gap repair was biased toward gene conversion events unassociated with crossing over using either donor sequence. The dependence of recombinational gap repair on genes belonging to the RAD52 epistasis group was tested in this system. RAD51, RAD52, RAD57, and RAD59 were required for efficient gap repair using either chromosomal or plasmid donors. No homologous recombination products were recovered from rad52 mutants, whereas a low level of repair occurred in the absence of RAD51, RAD57, or RAD59. These results suggest a minor pathway of strand invasion that is dependent on RAD52 but not on RAD51. The residual repair events in rad51 mutants were more frequently associated with crossing over than was observed in the wild-type strain, suggesting that the mechanisms for RAD51-dependent and RAD51-independent events are different. Plasmid gap repair was reduced synergistically in rad51 rad59 double mutants, indicating an important role for RAD59 in RAD51-independent repair.
Figures
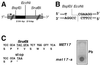
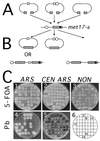
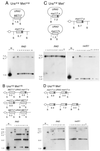
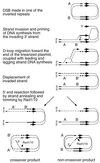
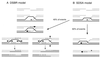
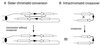
Similar articles
-
A Rad52 homolog is required for RAD51-independent mitotic recombination in Saccharomyces cerevisiae.Genes Dev. 1996 Aug 15;10(16):2025-37. doi: 10.1101/gad.10.16.2025. Genes Dev. 1996. PMID: 8769646
-
Genetic evidence for different RAD52-dependent intrachromosomal recombination pathways in Saccharomyces cerevisiae.Curr Genet. 1995 Mar;27(4):298-305. doi: 10.1007/BF00352096. Curr Genet. 1995. PMID: 7614550
-
Aberrant double-strand break repair in rad51 mutants of Saccharomyces cerevisiae.Mol Cell Biol. 2000 Dec;20(24):9162-72. doi: 10.1128/MCB.20.24.9162-9172.2000. Mol Cell Biol. 2000. PMID: 11094068 Free PMC article.
-
Recombination proteins in yeast.Annu Rev Genet. 2004;38:233-71. doi: 10.1146/annurev.genet.38.072902.091500. Annu Rev Genet. 2004. PMID: 15568977 Review.
-
Role of RAD52 epistasis group genes in homologous recombination and double-strand break repair.Microbiol Mol Biol Rev. 2002 Dec;66(4):630-70, table of contents. doi: 10.1128/MMBR.66.4.630-670.2002. Microbiol Mol Biol Rev. 2002. PMID: 12456786 Free PMC article. Review.
Cited by
-
The yeast Fun30 and human SMARCAD1 chromatin remodellers promote DNA end resection.Nature. 2012 Sep 27;489(7417):581-4. doi: 10.1038/nature11353. Epub 2012 Sep 9. Nature. 2012. PMID: 22960744 Free PMC article.
-
Different genetic requirements for repair of replication-born double-strand breaks by sister-chromatid recombination and break-induced replication.Nucleic Acids Res. 2007;35(19):6560-70. doi: 10.1093/nar/gkm488. Epub 2007 Sep 28. Nucleic Acids Res. 2007. PMID: 17905819 Free PMC article.
-
PCRless library mutagenesis via oligonucleotide recombination in yeast.Protein Sci. 2010 Dec;19(12):2336-46. doi: 10.1002/pro.513. Protein Sci. 2010. PMID: 20936671 Free PMC article.
-
A mechanism of gene amplification driven by small DNA fragments.PLoS Genet. 2012;8(12):e1003119. doi: 10.1371/journal.pgen.1003119. Epub 2012 Dec 13. PLoS Genet. 2012. PMID: 23271978 Free PMC article.
-
Targeted DNA integration within different functional gene domains in yeast reveals ORF sequences as recombinational cold-spots.Mol Genet Genomics. 2004 May;271(4):437-46. doi: 10.1007/s00438-004-0994-8. Epub 2004 Mar 27. Mol Genet Genomics. 2004. PMID: 15048565
References
-
- Aguilera A. Genetic evidence for different RAD52-dependent intrachromosomal recombination pathways in Saccharomyces cerevisiae. Curr Genet. 1995;27:298–305. - PubMed
-
- Allen J B, Zhou Z, Siede W, Friedberg E C, Elledge S J. The SAD1/RAD53 protein kinase controls multiple checkpoints and DNA damage-induced transcription in yeast. Genes Dev. 1994;8:2401–2415. - PubMed
-
- Bai Y, Symington L S. A Rad52 homolog is required for RAD51-independent mitotic recombination in Saccharomyces cerevisiae. Genes Dev. 1996;10:2025–2037. - PubMed
Publication types
MeSH terms
Substances
Grants and funding
LinkOut - more resources
Full Text Sources
Other Literature Sources
Molecular Biology Databases
Research Materials
Miscellaneous