Molecular tectonic model of virus structural transitions: the putative cell entry states of poliovirus
- PMID: 10627545
- PMCID: PMC111469
- DOI: 10.1128/jvi.74.3.1342-1354.2000
Molecular tectonic model of virus structural transitions: the putative cell entry states of poliovirus
Abstract
Upon interacting with its receptor, poliovirus undergoes conformational changes that are implicated in cell entry, including the externalization of the viral protein VP4 and the N terminus of VP1. We have determined the structures of native virions and of two putative cell entry intermediates, the 135S and 80S particles, at approximately 22-A resolution by cryo-electron microscopy. The 135S and 80S particles are both approximately 4% larger than the virion. Pseudoatomic models were constructed by adjusting the beta-barrel domains of the three capsid proteins VP1, VP2, and VP3 from their known positions in the virion to fit the 135S and 80S reconstructions. Domain movements of up to 9 A were detected, analogous to the shifting of tectonic plates. These movements create gaps between adjacent subunits. The gaps at the sites where VP1, VP2, and VP3 subunits meet are plausible candidates for the emergence of VP4 and the N terminus of VP1. The implications of these observations are discussed for models in which the externalized components form a transmembrane pore through which viral RNA enters the infected cell.
Figures
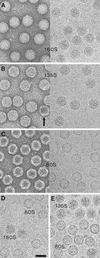
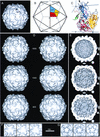
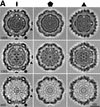
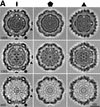
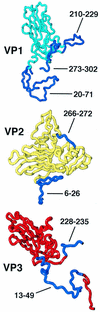
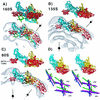
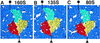
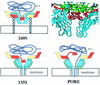
Similar articles
-
The structure of the poliovirus 135S cell entry intermediate at 10-angstrom resolution reveals the location of an externalized polypeptide that binds to membranes.J Virol. 2005 Jun;79(12):7745-55. doi: 10.1128/JVI.79.12.7745-7755.2005. J Virol. 2005. PMID: 15919927 Free PMC article.
-
An externalized polypeptide partitions between two distinct sites on genome-released poliovirus particles.J Virol. 2011 Oct;85(19):9974-83. doi: 10.1128/JVI.05013-11. Epub 2011 Jul 20. J Virol. 2011. PMID: 21775460 Free PMC article.
-
Cryo-electron microscopy reconstruction shows poliovirus 135S particles poised for membrane interaction and RNA release.J Virol. 2014 Feb;88(3):1758-70. doi: 10.1128/JVI.01949-13. Epub 2013 Nov 20. J Virol. 2014. PMID: 24257617 Free PMC article.
-
Poliovirus cell entry: common structural themes in viral cell entry pathways.Annu Rev Microbiol. 2002;56:677-702. doi: 10.1146/annurev.micro.56.012302.160757. Epub 2002 Jan 30. Annu Rev Microbiol. 2002. PMID: 12142481 Free PMC article. Review.
-
Beyond structures of highly symmetric purified viral capsids by cryo-EM.Curr Opin Struct Biol. 2018 Oct;52:25-31. doi: 10.1016/j.sbi.2018.07.011. Epub 2018 Aug 7. Curr Opin Struct Biol. 2018. PMID: 30096461 Review.
Cited by
-
Cryo-EM structures reveal two distinct conformational states in a picornavirus cell entry intermediate.PLoS Pathog. 2020 Sep 30;16(9):e1008920. doi: 10.1371/journal.ppat.1008920. eCollection 2020 Sep. PLoS Pathog. 2020. PMID: 32997730 Free PMC article.
-
ICAM-1 induced rearrangements of capsid and genome prime rhinovirus 14 for activation and uncoating.Proc Natl Acad Sci U S A. 2021 May 11;118(19):e2024251118. doi: 10.1073/pnas.2024251118. Proc Natl Acad Sci U S A. 2021. PMID: 33947819 Free PMC article.
-
All-atom multiscale simulation of cowpea chlorotic mottle virus capsid swelling.J Phys Chem B. 2010 Sep 2;114(34):11181-95. doi: 10.1021/jp102314e. J Phys Chem B. 2010. PMID: 20695471 Free PMC article.
-
Three-dimensional structure of poliovirus receptor bound to poliovirus.Proc Natl Acad Sci U S A. 2000 Jan 4;97(1):73-8. doi: 10.1073/pnas.97.1.73. Proc Natl Acad Sci U S A. 2000. PMID: 10618373 Free PMC article.
-
Mutation in loop I of VP1 of Theiler's virus delays viral RNA release into cells and enhances antibody-mediated neutralization: a mechanism for the failure of persistence by the mutant virus.J Neurovirol. 2002 Apr;8(2):100-10. doi: 10.1080/13550280290049561. J Neurovirol. 2002. PMID: 11935462
References
-
- Baker T S, Cheng R H. A model-based approach for determining orientations of biological macromolecules imaged by cryo-electron microscopy. J Struct Biol. 1996;116:120–130. - PubMed
Publication types
MeSH terms
Substances
Grants and funding
LinkOut - more resources
Full Text Sources
Other Literature Sources