Dynein intermediate chain mediated dynein-dynactin interaction is required for interphase microtubule organization and centrosome replication and separation in Dictyostelium
- PMID: 10601339
- PMCID: PMC2168085
- DOI: 10.1083/jcb.147.6.1261
Dynein intermediate chain mediated dynein-dynactin interaction is required for interphase microtubule organization and centrosome replication and separation in Dictyostelium
Abstract
Cytoplasmic dynein intermediate chain (IC) mediates dynein-dynactin interaction in vitro (Karki, S., and E.L. Holzbaur. 1995. J. Biol. Chem. 270:28806-28811; Vaughan, K.T., and R.B. Vallee. 1995. J. Cell Biol. 131:1507-1516). To investigate the physiological role of IC and dynein-dynactin interaction, we expressed IC truncations in wild-type Dictyostelium cells. ICDeltaC associated with dynactin but not with dynein heavy chain, whereas ICDeltaN truncations bound to dynein but bound dynactin poorly. Both mutations resulted in abnormal localization to the Golgi complex, confirming dynein function was disrupted. Striking disorganization of interphase microtubule (MT) networks was observed when mutant expression was induced. In a majority of cells, the MT networks collapsed into large bundles. We also observed cells with multiple cytoplasmic asters and MTs lacking an organizing center. These cells accumulated abnormal DNA content, suggesting a defect in mitosis. Striking defects in centrosome morphology were also observed in IC mutants, mostly larger than normal centrosomes. Ultrastructural analysis of centrosomes in IC mutants showed interphase accumulation of large centrosomes typical of prophase as well as unusually paired centrosomes, suggesting defects in centrosome replication and separation. These results suggest that dynactin-mediated cytoplasmic dynein function is required for the proper organization of interphase MT network as well as centrosome replication and separation in Dictyostelium.
Figures
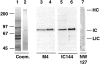
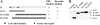
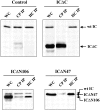
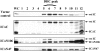
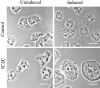
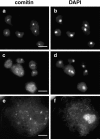
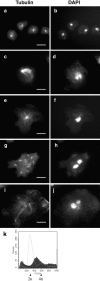
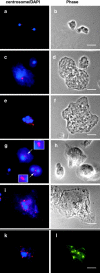
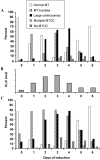
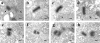
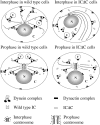
Similar articles
-
Dynactin is required for microtubule anchoring at centrosomes.J Cell Biol. 1999 Oct 18;147(2):321-34. doi: 10.1083/jcb.147.2.321. J Cell Biol. 1999. PMID: 10525538 Free PMC article.
-
Cytoplasmic dynein is involved in the retention of microtubules at the centrosome in interphase cells.Traffic. 2008 Apr;9(4):472-80. doi: 10.1111/j.1600-0854.2007.00698.x. Epub 2007 Dec 26. Traffic. 2008. PMID: 18182007
-
[Dynein and dynactin as organizers of the system of cell microtubules].Ontogenez. 2006 Sep-Oct;37(5):323-39. Ontogenez. 2006. PMID: 17066975 Review. Russian.
-
Direct interaction of pericentrin with cytoplasmic dynein light intermediate chain contributes to mitotic spindle organization.J Cell Biol. 1999 Nov 1;147(3):481-92. doi: 10.1083/jcb.147.3.481. J Cell Biol. 1999. PMID: 10545494 Free PMC article.
-
Role of NuMA in vertebrate cells: review of an intriguing multifunctional protein.Front Biosci. 2006 Jan 1;11:1137-46. doi: 10.2741/1868. Front Biosci. 2006. PMID: 16146802 Review.
Cited by
-
Dynein light intermediate chain in Aspergillus nidulans is essential for the interaction between heavy and intermediate chains.J Biol Chem. 2009 Dec 11;284(50):34760-8. doi: 10.1074/jbc.M109.026872. Epub 2009 Oct 16. J Biol Chem. 2009. PMID: 19837669 Free PMC article.
-
Pushing forces drive the comet-like motility of microtubule arrays in Dictyostelium.Mol Biol Cell. 2005 Jul;16(7):3334-40. doi: 10.1091/mbc.e05-01-0057. Epub 2005 Apr 27. Mol Biol Cell. 2005. PMID: 15857957 Free PMC article.
-
A molecular genetic analysis of the interaction between the cytoplasmic dynein intermediate chain and the glued (dynactin) complex.Mol Biol Cell. 2000 Nov;11(11):3791-803. doi: 10.1091/mbc.11.11.3791. Mol Biol Cell. 2000. PMID: 11071907 Free PMC article.
-
On and off controls within dynein-dynactin on native cargoes.Proc Natl Acad Sci U S A. 2021 Jun 8;118(23):e2103383118. doi: 10.1073/pnas.2103383118. Proc Natl Acad Sci U S A. 2021. PMID: 34074788 Free PMC article.
-
Force balances between interphase centrosomes as revealed by laser ablation.Mol Biol Cell. 2019 Jul 1;30(14):1705-1715. doi: 10.1091/mbc.E19-01-0034. Epub 2019 May 8. Mol Biol Cell. 2019. PMID: 31067156 Free PMC article.
References
-
- Boleti H., Karsenti E., Vernos I. Xklp2, a novel Xenopus centrosomal kinesin-like protein required for centrosome separation during mitosis. Cell. 1996;84:49–59. - PubMed
-
- Bomsel M., Parton R., Kuznetsov S.A., Schroer T.A., Gruenberg J. Microtubule- and motor-dependent fusion in vitro between apical and basolateral endocytic vesicles from MDCK cells. Cell. 1990;62:719–731. - PubMed
Publication types
MeSH terms
Substances
Grants and funding
LinkOut - more resources
Full Text Sources
Molecular Biology Databases