Dynamin-dependent endocytosis of ionotropic glutamate receptors
- PMID: 10570207
- PMCID: PMC24199
- DOI: 10.1073/pnas.96.24.14112
Dynamin-dependent endocytosis of ionotropic glutamate receptors
Abstract
Little is known about the mechanisms that regulate the number of ionotropic glutamate receptors present at excitatory synapses. Herein, we show that GluR1-containing alpha-amino-3-hydroxy-5-methyl-4-isoxazolepropionic acid (AMPA) receptors (AMPARs) are removed from the postsynaptic plasma membrane of cultured hippocampal neurons by rapid, ligand-induced endocytosis. Although endocytosis of AMPARs can be induced by high concentrations of AMPA without concomitant activation of N-methyl-D-aspartate (NMDA) receptors (NMDARs), NMDAR activation is required for detectable endocytosis induced by synaptically released glutamate. Activated AMPARs colocalize with AP2, a marker of endocytic coated pits, and endocytosis of AMPARs is blocked by biochemical inhibition of clathrin-coated pit function or overexpression of a dominant-negative mutant form of dynamin. These results establish that ionotropic receptors are regulated by dynamin-dependent endocytosis and suggest an important role of endocytic membrane trafficking in the postsynaptic modulation of neurotransmission.
Figures
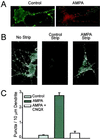
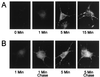
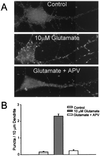
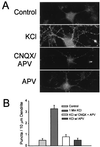
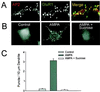
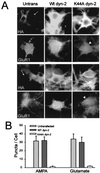
Similar articles
-
Constitutive endocytosis of GABAA receptors by an association with the adaptin AP2 complex modulates inhibitory synaptic currents in hippocampal neurons.J Neurosci. 2000 Nov 1;20(21):7972-7. doi: 10.1523/JNEUROSCI.20-21-07972.2000. J Neurosci. 2000. PMID: 11050117 Free PMC article.
-
Subunit dependencies of N-methyl-D-aspartate (NMDA) receptor-induced alpha-amino-3-hydroxy-5-methyl-4-isoxazolepropionic acid (AMPA) receptor internalization.Mol Pharmacol. 2006 Apr;69(4):1251-9. doi: 10.1124/mol.105.018580. Epub 2006 Jan 25. Mol Pharmacol. 2006. PMID: 16436589
-
Regulation of AMPA receptor-mediated synaptic transmission by clathrin-dependent receptor internalization.Neuron. 2000 Mar;25(3):649-62. doi: 10.1016/s0896-6273(00)81067-3. Neuron. 2000. PMID: 10774732
-
The mechanistic link between Arc/Arg3.1 expression and AMPA receptor endocytosis.Semin Cell Dev Biol. 2018 May;77:17-24. doi: 10.1016/j.semcdb.2017.09.005. Epub 2017 Sep 7. Semin Cell Dev Biol. 2018. PMID: 28890421 Review.
-
AMPA and NMDA glutamate receptor trafficking: multiple roads for reaching and leaving the synapse.Cell Tissue Res. 2006 Nov;326(2):423-38. doi: 10.1007/s00441-006-0254-9. Epub 2006 Jul 18. Cell Tissue Res. 2006. PMID: 16847641 Review.
Cited by
-
Control of neuronal ion channel function by glycogen synthase kinase-3: new prospective for an old kinase.Front Mol Neurosci. 2012 Jul 16;5:80. doi: 10.3389/fnmol.2012.00080. eCollection 2012. Front Mol Neurosci. 2012. PMID: 22811658 Free PMC article.
-
Calcium-permeable AMPA receptors are expressed in a rodent model of status epilepticus.Ann Neurol. 2012 Jul;72(1):91-102. doi: 10.1002/ana.23570. Ann Neurol. 2012. PMID: 22829271 Free PMC article.
-
Real-time imaging of discrete exocytic events mediating surface delivery of AMPA receptors.J Neurosci. 2007 Oct 10;27(41):11112-21. doi: 10.1523/JNEUROSCI.2465-07.2007. J Neurosci. 2007. PMID: 17928453 Free PMC article.
-
Arc/Arg3.1 interacts with the endocytic machinery to regulate AMPA receptor trafficking.Neuron. 2006 Nov 9;52(3):445-59. doi: 10.1016/j.neuron.2006.08.033. Neuron. 2006. PMID: 17088211 Free PMC article.
-
Intracellular machinery for the transport of AMPA receptors.Br J Pharmacol. 2008 Mar;153 Suppl 1(Suppl 1):S35-43. doi: 10.1038/sj.bjp.0707525. Epub 2007 Nov 19. Br J Pharmacol. 2008. PMID: 18026130 Free PMC article. Review.
References
-
- Hollmann M, Heinemann S. Annu Rev Neurosci. 1994;17:31–108. - PubMed
-
- O’Brien R J, Lau L F, Huganir R L. Curr Opin Neurobiol. 1998;8:364–369. - PubMed
-
- Seeburg P H. Trends Neurosci. 1993;16:359–365. - PubMed
-
- Gomperts S N, Rao A, Craig A M, Malenka R C, Nicoll R A. Neuron. 1998;21:1443–1451. - PubMed
-
- Malenka R C, Nicoll R A. Neuron. 1997;19:473–476. - PubMed
Publication types
MeSH terms
Substances
LinkOut - more resources
Full Text Sources
Other Literature Sources
Research Materials