Chaperone rings in protein folding and degradation
- PMID: 10500119
- PMCID: PMC34237
- DOI: 10.1073/pnas.96.20.11033
Chaperone rings in protein folding and degradation
Abstract
Chaperone rings play a vital role in the opposing ATP-mediated processes of folding and degradation of many cellular proteins, but the mechanisms by which they assist these life and death actions are only beginning to be understood. Ring structures present an advantage to both processes, providing for compartmentalization of the substrate protein inside a central cavity in which multivalent, potentially cooperative interactions can take place between the substrate and a high local concentration of binding sites, while access of other proteins to the cavity is restricted sterically. Such restriction prevents outside interference that could lead to nonproductive fates of the substrate protein while it is present in non-native form, such as aggregation. At the step of recognition, chaperone rings recognize different motifs in their substrates, exposed hydrophobicity in the case of protein-folding chaperonins, and specific "tag" sequences in at least some cases of the proteolytic chaperones. For both folding and proteolytic complexes, ATP directs conformational changes in the chaperone rings that govern release of the bound polypeptide. In the case of chaperonins, ATP enables a released protein to pursue the native state in a sequestered hydrophilic folding chamber, and, in the case of the proteases, the released polypeptide is translocated into a degradation chamber. These divergent fates are at least partly governed by very different cooperating components that associate with the chaperone rings: that is, cochaperonin rings on one hand and proteolytic ring assemblies on the other. Here we review the structures and mechanisms of the two types of chaperone ring system.
Figures
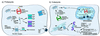
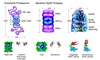
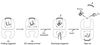
Similar articles
-
Folding on the chaperone: yield enhancement through loose binding.J Mol Biol. 2006 Nov 10;363(5):945-57. doi: 10.1016/j.jmb.2006.08.040. Epub 2006 Aug 22. J Mol Biol. 2006. PMID: 16987526
-
Review: a structural view of the GroE chaperone cycle.J Struct Biol. 2001 Aug;135(2):95-103. doi: 10.1006/jsbi.2001.4387. J Struct Biol. 2001. PMID: 11580259 Review.
-
Roles of molecular chaperones in cytoplasmic protein folding.Semin Cell Dev Biol. 2000 Feb;11(1):15-25. doi: 10.1006/scdb.1999.0347. Semin Cell Dev Biol. 2000. PMID: 10736260 Review.
-
GroEL/GroES: structure and function of a two-stroke folding machine.J Struct Biol. 1998 Dec 15;124(2-3):129-41. doi: 10.1006/jsbi.1998.4060. J Struct Biol. 1998. PMID: 10049801 Review.
-
Chaperonin-mediated protein folding: fate of substrate polypeptide.Q Rev Biophys. 2003 May;36(2):229-56. doi: 10.1017/s0033583503003883. Q Rev Biophys. 2003. PMID: 14686103 Review.
Cited by
-
Protein unfolding by mitochondria. The Hsp70 import motor.EMBO Rep. 2000 Nov;1(5):404-10. doi: 10.1093/embo-reports/kvd093. EMBO Rep. 2000. PMID: 11258479 Free PMC article. Review.
-
Intracellular aggregation of polypeptides with expanded polyglutamine domain is stimulated by stress-activated kinase MEKK1.J Cell Biol. 2001 May 14;153(4):851-64. doi: 10.1083/jcb.153.4.851. J Cell Biol. 2001. PMID: 11352944 Free PMC article.
-
The ubiquitin-proteasome system in retinal health and disease.Mol Neurobiol. 2013 Apr;47(2):790-810. doi: 10.1007/s12035-012-8391-5. Epub 2013 Jan 22. Mol Neurobiol. 2013. PMID: 23339020 Review.
-
Two peptide sequences can function cooperatively to facilitate binding and unfolding by ClpA and degradation by ClpAP.Proc Natl Acad Sci U S A. 2006 Jan 24;103(4):909-14. doi: 10.1073/pnas.0509154103. Epub 2006 Jan 12. Proc Natl Acad Sci U S A. 2006. PMID: 16410355 Free PMC article.
-
ClpC2 protects mycobacteria against a natural antibiotic targeting ClpC1-dependent protein degradation.Commun Biol. 2023 Mar 21;6(1):301. doi: 10.1038/s42003-023-04658-9. Commun Biol. 2023. PMID: 36944713 Free PMC article.
References
-
- Baumeister W, Walz J, Zühl, Seemüller E. Cell. 1998;92:367–380. - PubMed
-
- Bukau B, Horwich A L. Cell. 1988;92:351–366. - PubMed
-
- Xu Z, Horwich A L, Sigler P B. Nature (London) 1997;388:741–750. - PubMed
-
- Ditzel L, Löwe J, Stock D, Stetter K-O, Huber H, Huber R, Steinbacher S. Cell. 1998;93:125–138. - PubMed
-
- Lewis V A, Hynes G M, Zheng D, Saibil H, Willison K. Nature (London) 1992;358:249–252. - PubMed
Publication types
MeSH terms
Substances
LinkOut - more resources
Full Text Sources