A Streptomyces coelicolor antibiotic regulatory gene, absB, encodes an RNase III homolog
- PMID: 10498729
- PMCID: PMC103644
- DOI: 10.1128/JB.181.19.6142-6151.1999
A Streptomyces coelicolor antibiotic regulatory gene, absB, encodes an RNase III homolog
Abstract
Streptomyces coelicolor produces four genetically and structurally distinct antibiotics in a growth-phase-dependent manner. S. coelicolor mutants globally deficient in antibiotic production (Abs(-) phenotype) have previously been isolated, and some of these were found to define the absB locus. In this study, we isolated absB-complementing DNA and show that it encodes the S. coelicolor homolog of RNase III (rnc). Several lines of evidence indicate that the absB mutant global defect in antibiotic synthesis is due to a deficiency in RNase III. In marker exchange experiments, the S. coelicolor rnc gene rescued absB mutants, restoring antibiotic production. Sequencing the DNA of absB mutants confirmed that the absB mutations lay in the rnc open reading frame. Constructed disruptions of rnc in both S. coelicolor 1501 and Streptomyces lividans 1326 caused an Abs(-) phenotype. An absB mutation caused accumulation of 30S rRNA precursors, as had previously been reported for E. coli rnc mutants. The absB gene is widely conserved in streptomycetes. We speculate on why an RNase III deficiency could globally affect the synthesis of antibiotics.
Figures
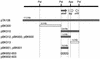
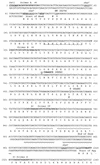
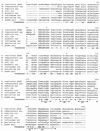
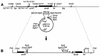
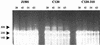
Similar articles
-
Transcriptional regulation of Streptomyces coelicolor pathway-specific antibiotic regulators by the absA and absB loci.J Bacteriol. 1998 Jun;180(12):3100-6. doi: 10.1128/JB.180.12.3100-3106.1998. J Bacteriol. 1998. PMID: 9620958 Free PMC article.
-
Genetic analysis of absB, a Streptomyces coelicolor locus involved in global antibiotic regulation.J Bacteriol. 1992 Jul;174(14):4622-8. doi: 10.1128/jb.174.14.4622-4628.1992. J Bacteriol. 1992. PMID: 1624449 Free PMC article.
-
The absB gene encodes a double strand-specific endoribonuclease that cleaves the read-through transcript of the rpsO-pnp operon in Streptomyces coelicolor.J Biol Chem. 2005 Sep 30;280(39):33213-9. doi: 10.1074/jbc.M503440200. Epub 2005 Aug 1. J Biol Chem. 2005. PMID: 16076842
-
RNA degradation and the regulation of antibiotic synthesis in Streptomyces.Future Microbiol. 2010 Mar;5(3):419-29. doi: 10.2217/fmb.10.14. Future Microbiol. 2010. PMID: 20210552 Review.
-
Genetics of antibiotic production in Streptomyces coelicolor A3(2), a model streptomycete.Biotechnology. 1995;28:65-102. doi: 10.1016/b978-0-7506-9095-9.50009-5. Biotechnology. 1995. PMID: 8688641 Review. No abstract available.
Cited by
-
An oligoribonuclease gene in Streptomyces griseus.J Bacteriol. 2000 Aug;182(16):4647-53. doi: 10.1128/JB.182.16.4647-4653.2000. J Bacteriol. 2000. PMID: 10913103 Free PMC article.
-
RNase III is required for actinomycin production in Streptomyces antibioticus.Appl Environ Microbiol. 2013 Oct;79(20):6447-51. doi: 10.1128/AEM.02272-13. Epub 2013 Aug 16. Appl Environ Microbiol. 2013. PMID: 23956389 Free PMC article.
-
Streptomyces RNases - Function and impact on antibiotic synthesis.Front Microbiol. 2023 Apr 11;14:1096228. doi: 10.3389/fmicb.2023.1096228. eCollection 2023. Front Microbiol. 2023. PMID: 37113221 Free PMC article. Review.
-
SCO5745, a bifunctional RNase J ortholog, affects antibiotic production in Streptomyces coelicolor.J Bacteriol. 2014 Mar;196(6):1197-205. doi: 10.1128/JB.01422-13. Epub 2014 Jan 10. J Bacteriol. 2014. PMID: 24415725 Free PMC article.
-
Phosphorylated AbsA2 negatively regulates antibiotic production in Streptomyces coelicolor through interactions with pathway-specific regulatory gene promoters.J Bacteriol. 2007 Jul;189(14):5284-92. doi: 10.1128/JB.00305-07. Epub 2007 May 18. J Bacteriol. 2007. PMID: 17513473 Free PMC article.
References
-
- Adamidis A. Ph.D. dissertation. East Lansing: Michigan State University; 1994.
Publication types
MeSH terms
Substances
LinkOut - more resources
Full Text Sources
Medical