Unraveling a cytoplasmic role for hnRNP D in the in vivo mRNA destabilization directed by the AU-rich element
- PMID: 10421639
- PMCID: PMC316883
- DOI: 10.1101/gad.13.14.1884
Unraveling a cytoplasmic role for hnRNP D in the in vivo mRNA destabilization directed by the AU-rich element
Abstract
AU-rich RNA-destabilizing elements (AREs) have become a paradigm for studying cytoplasmic mRNA turnover in mammalian cells. Though many RNA-binding proteins have been shown to bind to AREs in vitro, trans-acting factors that participate in the in vivo destabilization of cytoplasmic RNA by AREs remains unknown. Experiments were performed to investigate the cellular mechanisms and to identify potential trans-acting factors for ARE-directed mRNA decay. These experiments identified hnRNP D, a heterogeneous nuclear ribonucleoprotein (hnRNP) capable of shuttling between the nucleus and cytoplasm, as an RNA destabilizing protein in vivo in ARE-mediated rapid mRNA decay. Our results show that the ARE destabilizing function is dramatically impeded during hemin-induced erythroid differentiation and not in TPA-induced megakaryocytic differentiation of human erythroleukemic K562 cells. A sequestration of hnRNP D into a hemin-induced protein complex, termed hemin-regulated factor or HRF, correlates well with the loss of ARE-destabilizing function in the cytoplasm. Further experiments show that in hemin-treated cells, ectopic expression of hnRNP D restores the rapid decay directed by the ARE. The extent of destabilizing effect varies among the four isoforms of hnRNP D, with p37 and p42 displaying the most profound effect. These results demonstrate a specific cytoplasmic function for hnRNP D as an RNA-destabilizing protein in ARE-mediated decay pathway. These in vivo findings support an emerging idea that shuttling hnRNP proteins have not only a nuclear but also a cytoplasmic function in mRNA metabolism. The data further imply that shuttling hnRNP proteins define, at least in part, the nuclear history of individual mRNAs and thereby influence their cytoplasmic fate.
Figures
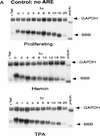
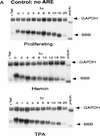
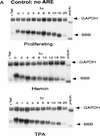
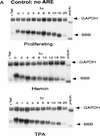
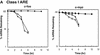
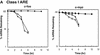
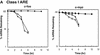
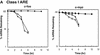
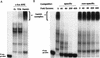
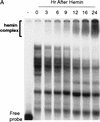
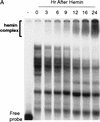
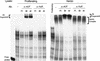
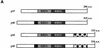
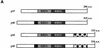
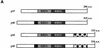
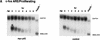
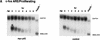
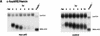
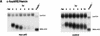
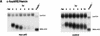
Similar articles
-
Versatile role for hnRNP D isoforms in the differential regulation of cytoplasmic mRNA turnover.Mol Cell Biol. 2001 Oct;21(20):6960-71. doi: 10.1128/MCB.21.20.6960-6971.2001. Mol Cell Biol. 2001. PMID: 11564879 Free PMC article.
-
Modulation of AUUUA response element binding by heterogeneous nuclear ribonucleoprotein A1 in human T lymphocytes. The roles of cytoplasmic location, transcription, and phosphorylation.J Biol Chem. 1997 Nov 7;272(45):28732-41. doi: 10.1074/jbc.272.45.28732. J Biol Chem. 1997. PMID: 9353343
-
AU-A, an RNA-binding activity distinct from hnRNP A1, is selective for AUUUA repeats and shuttles between the nucleus and the cytoplasm.Nucleic Acids Res. 1994 Jan 25;22(2):238-46. doi: 10.1093/nar/22.2.238. Nucleic Acids Res. 1994. PMID: 8121809 Free PMC article.
-
Heterogeneous nuclear ribonucleoproteins (hnRNPs) in cellular processes: Focus on hnRNP E1's multifunctional regulatory roles.RNA. 2010 Aug;16(8):1449-62. doi: 10.1261/rna.2254110. Epub 2010 Jun 28. RNA. 2010. PMID: 20584894 Free PMC article. Review.
-
Functional diversity of hnRNP proteins.Indian J Biochem Biophys. 2001 Jun;38(3):129-34. Indian J Biochem Biophys. 2001. PMID: 11693373 Review.
Cited by
-
hnRNPs: roles in neurodevelopment and implication for brain disorders.Front Mol Neurosci. 2024 Jul 17;17:1411639. doi: 10.3389/fnmol.2024.1411639. eCollection 2024. Front Mol Neurosci. 2024. PMID: 39086926 Free PMC article. Review.
-
Cellular mutants define a common mRNA degradation pathway targeting cytokine AU-rich elements.RNA. 2001 Nov;7(11):1578-88. RNA. 2001. PMID: 11720287 Free PMC article.
-
Tethering KSRP, a decay-promoting AU-rich element-binding protein, to mRNAs elicits mRNA decay.Mol Cell Biol. 2006 May;26(10):3695-706. doi: 10.1128/MCB.26.10.3695-3706.2006. Mol Cell Biol. 2006. PMID: 16648466 Free PMC article.
-
AU-rich elements and associated factors: are there unifying principles?Nucleic Acids Res. 2006 Jan 3;33(22):7138-50. doi: 10.1093/nar/gki1012. Print 2005. Nucleic Acids Res. 2006. PMID: 16391004 Free PMC article. Review.
-
Selective degradation of AU-rich mRNAs promoted by the p37 AUF1 protein isoform.Mol Cell Biol. 2003 Sep;23(18):6685-93. doi: 10.1128/MCB.23.18.6685-6693.2003. Mol Cell Biol. 2003. PMID: 12944492 Free PMC article.
References
-
- Alitalo R. Induced differentiation of K562 leukemia cells: A model for studies of gene expression in early megakaryoblasts. Leuk Res. 1990;14:501–514. - PubMed
-
- Alitalo R, Mäkelä TP, Koskinen P, Andersson LC, Alitalo K. Enhanced expression of transforming growth factor beta during megakaryoblastic differentiation of K562 leukemia cells. Blood. 1988;71:899–906. - PubMed
-
- Baliga BS, Mankad M, Shah AK, Mankad VN. Mechanism of differentiation of human erythroleukaemic cell line K562 by hemin. Cell Prolif. 1993;26:519–529. - PubMed
Publication types
MeSH terms
Substances
Grants and funding
LinkOut - more resources
Full Text Sources