Replication-competent rhabdoviruses with human immunodeficiency virus type 1 coats and green fluorescent protein: entry by a pH-independent pathway
- PMID: 10400792
- PMCID: PMC112779
- DOI: 10.1128/JVI.73.8.6937-6945.1999
Replication-competent rhabdoviruses with human immunodeficiency virus type 1 coats and green fluorescent protein: entry by a pH-independent pathway
Abstract
We describe a replication-competent, recombinant vesicular stomatitis virus (VSV) in which the gene encoding the single transmembrane glycoprotein (G) was deleted and replaced by an env-G hybrid gene encoding the extracellular and transmembrane domains of a human immunodeficiency virus type 1 (HIV-1) envelope protein fused to the cytoplasmic domain of VSV G. An additional gene encoding a green fluorescent protein was added to permit rapid detection of infection. This novel surrogate virus infected and propagated on cells expressing the HIV receptor CD4 and coreceptor CXCR4. Infection was blocked by SDF-1, the ligand for CXCR4, by antibody to CD4 and by HIV-neutralizing antibody. This virus, unlike VSV, entered cells by a pH-independent pathway and thus supports a pH-independent pathway of HIV entry. Additional recombinants carrying hybrid env-G genes derived from R5 or X4R5 HIV strains also showed the coreceptor specificities of the HIV strains from which they were derived. These surrogate viruses provide a simple and rapid assay for HIV-neutralizing antibodies as well as a rapid screen for molecules that would interfere with any stage of HIV binding or entry. The viruses might also be useful as HIV vaccines. Our results suggest wide applications of other surrogate viruses based on VSV.
Figures
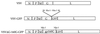
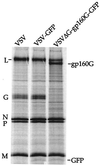
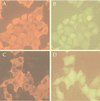
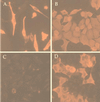
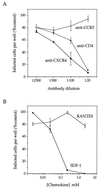
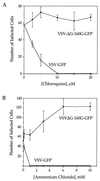
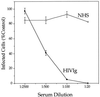
Similar articles
-
Specific targeting to CD4+ cells of recombinant vesicular stomatitis viruses encoding human immunodeficiency virus envelope proteins.J Virol. 1997 Jul;71(7):5060-8. doi: 10.1128/JVI.71.7.5060-5068.1997. J Virol. 1997. PMID: 9188571 Free PMC article.
-
Mutagenesis of CXCR4 identifies important domains for human immunodeficiency virus type 1 X4 isolate envelope-mediated membrane fusion and virus entry and reveals cryptic coreceptor activity for R5 isolates.J Virol. 1999 Aug;73(8):6598-609. doi: 10.1128/JVI.73.8.6598-6609.1999. J Virol. 1999. PMID: 10400757 Free PMC article.
-
A recombinant vesicular stomatitis virus encoding CCR5-tropic HIV-1 receptors targets HIV-1-infected cells and controls HIV-1 infection.Microbes Infect. 2017 Apr-May;19(4-5):277-287. doi: 10.1016/j.micinf.2016.12.004. Epub 2016 Dec 24. Microbes Infect. 2017. PMID: 28025070
-
Coreceptor usage of BOB/GPR15 and Bonzo/STRL33 by primary isolates of human immunodeficiency virus type 1.J Gen Virol. 1999 May;80 ( Pt 5):1241-1251. doi: 10.1099/0022-1317-80-5-1241. J Gen Virol. 1999. PMID: 10355771
-
Human immunodeficiency virus type 1 uses lipid raft-colocalized CD4 and chemokine receptors for productive entry into CD4(+) T cells.J Virol. 2002 May;76(10):4709-22. doi: 10.1128/jvi.76.10.4709-4722.2002. J Virol. 2002. PMID: 11967288 Free PMC article.
Cited by
-
Thogoto virus lacking interferon-antagonistic protein ML is strongly attenuated in newborn Mx1-positive but not Mx1-negative mice.J Virol. 2004 Oct;78(20):11422-4. doi: 10.1128/JVI.78.20.11422-11424.2004. J Virol. 2004. PMID: 15452266 Free PMC article.
-
Role of N-linked glycans in a human immunodeficiency virus envelope glycoprotein: effects on protein function and the neutralizing antibody response.J Virol. 2002 May;76(9):4199-211. doi: 10.1128/jvi.76.9.4199-4211.2002. J Virol. 2002. PMID: 11932385 Free PMC article.
-
Chemogenetic ON and OFF switches for RNA virus replication.Nat Commun. 2021 Mar 1;12(1):1362. doi: 10.1038/s41467-021-21630-5. Nat Commun. 2021. PMID: 33649317 Free PMC article.
-
Experimental Evolution Generates Novel Oncolytic Vesicular Stomatitis Viruses with Improved Replication in Virus-Resistant Pancreatic Cancer Cells.J Virol. 2020 Jan 17;94(3):e01643-19. doi: 10.1128/JVI.01643-19. Print 2020 Jan 17. J Virol. 2020. PMID: 31694943 Free PMC article.
-
A time-resolved molecular map of the macrophage response to VSV infection.NPJ Syst Biol Appl. 2016 Oct 27;2:16027. doi: 10.1038/npjsba.2016.27. eCollection 2016. NPJ Syst Biol Appl. 2016. PMID: 28725479 Free PMC article.
References
-
- Alkhatib G, Combadiere C, Broder C C, Feng Y, Kennedy P E, Murphy P M, Berger E A. CC CKR5: a RANTES, MIP-1alpha, MIP-1beta receptor as a fusion cofactor for macrophage-tropic HIV-1. Science. 1996;272:1955–1958. - PubMed
-
- Berger E A, Doms R W, Fenyo E M, Korber B T, Littman D R, Moore J P, Sattentau Q J, Schuitemaker H, Sodroski J, Weiss R A. A new classification for HIV-1. Nature. 1998;391:240. - PubMed
-
- Bleul C C, Farzan M, Choe H, Parolin C, Clark-Lewis I, Sodroski J, Springer T A. The lymphocyte chemoattractant SDF-1 is a ligand for LESTR/fusin and blocks HIV-1 entry. Nature. 1996;382:829–833. - PubMed
Publication types
MeSH terms
Substances
Grants and funding
LinkOut - more resources
Full Text Sources
Other Literature Sources
Research Materials