RNase P RNAs from some Archaea are catalytically active
- PMID: 10393902
- PMCID: PMC22142
- DOI: 10.1073/pnas.96.14.7803
RNase P RNAs from some Archaea are catalytically active
Abstract
The RNA subunits of RNase Ps of Archaea and eukaryotes have been thought to depend fundamentally on protein for activity, unlike those of Bacteria that are capable of efficient catalysis in the absence of protein. Although the eukaryotic RNase P RNAs are quite different than those of Bacteria in both sequence and structure, the archaeal RNAs generally contain the sequences and structures of the bacterial, phylogenetically conserved catalytic core. A spectrum of archaeal RNase P RNAs were therefore tested for activity in a wide range of conditions. Many remain inactive in ionically extreme conditions, but catalytic activity could be detected from those of the methanobacteria, thermococci, and halobacteria. Chimeric holoenzymes, reconstituted from the Methanobacterium RNase P RNA and the Bacillus subtilis RNase P protein subunits, were functional at low ionic strength. The properties of the archaeal RNase P RNAs (high ionic-strength requirement, low affinity for substrate, and catalytic reconstitution by bacterial RNase P protein) are similar to synthetic RNase P RNAs that contain all of the catalytic core of the bacterial RNA but lack phylogenetically variable, stabilizing elements.
Figures
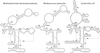
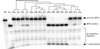
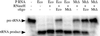
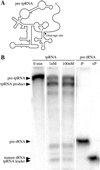
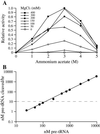
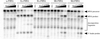
Similar articles
-
Archaeal RNase P has multiple protein subunits homologous to eukaryotic nuclear RNase P proteins.RNA. 2002 Mar;8(3):296-306. doi: 10.1017/s1355838202028492. RNA. 2002. PMID: 12003490 Free PMC article.
-
Comparative analysis of ribonuclease P RNA structure in Archaea.Nucleic Acids Res. 1996 Apr 1;24(7):1252-9. doi: 10.1093/nar/24.7.1252. Nucleic Acids Res. 1996. PMID: 8614627 Free PMC article.
-
In vitro selection of an archaeal RNase P RNA mimics natural variation.Archaea. 2004 Oct;1(4):241-5. doi: 10.1155/2004/903283. Archaea. 2004. PMID: 15810433 Free PMC article.
-
Ribonuclease P structure and function in Archaea.Mol Biol Rep. 1995-1996;22(2-3):131-4. doi: 10.1007/BF00988717. Mol Biol Rep. 1995. PMID: 8901499 Review.
-
RNase P RNA of Mycoplasma capricolum.Mol Biol Rep. 1995-1996;22(2-3):125-9. doi: 10.1007/BF00988716. Mol Biol Rep. 1995. PMID: 8901498 Review.
Cited by
-
Bacterial type B RNase P: functional characterization of the L5.1-L15.1 tertiary contact and antisense inhibition.RNA. 2016 Nov;22(11):1699-1709. doi: 10.1261/rna.057422.116. Epub 2016 Sep 7. RNA. 2016. PMID: 27604960 Free PMC article.
-
Interactions between subunits of Saccharomyces cerevisiae RNase MRP support a conserved eukaryotic RNase P/MRP architecture.Nucleic Acids Res. 2007;35(19):6439-50. doi: 10.1093/nar/gkm553. Epub 2007 Sep 19. Nucleic Acids Res. 2007. PMID: 17881380 Free PMC article.
-
Ribosomal protein L7Ae is a subunit of archaeal RNase P.Proc Natl Acad Sci U S A. 2010 Aug 17;107(33):14573-8. doi: 10.1073/pnas.1005556107. Epub 2010 Jul 30. Proc Natl Acad Sci U S A. 2010. PMID: 20675586 Free PMC article.
-
Archaeal/eukaryal RNase P: subunits, functions and RNA diversification.Nucleic Acids Res. 2010 Dec;38(22):7885-94. doi: 10.1093/nar/gkq701. Epub 2010 Aug 16. Nucleic Acids Res. 2010. PMID: 20716516 Free PMC article. Review.
-
Solution structure of an archaeal RNase P binary protein complex: formation of the 30-kDa complex between Pyrococcus furiosus RPP21 and RPP29 is accompanied by coupled protein folding and highlights critical features for protein-protein and protein-RNA interactions.J Mol Biol. 2009 Nov 13;393(5):1043-55. doi: 10.1016/j.jmb.2009.08.068. Epub 2009 Sep 3. J Mol Biol. 2009. PMID: 19733182 Free PMC article.
References
-
- Karwan R, Pluk H, van Venrooij W J, editors. Molecular Biology Reports Special Issue: RNase MRP/RNase P Systems. Vol. 22. Nijmegen: Kluwer; 1996.
-
- Reich C, Olsen G J, Pace B, Pace N R. Science. 1988;239:178–181. - PubMed
-
- Kurz J C, Niranjanakumari S, Fierke C A. Biochemistry. 1998;37:2393–2400. - PubMed
-
- Guerrier-Takada C, Gardner K, Marsh T, Pace N R, Altman S. Cell. 1983;35:849–857. - PubMed
Publication types
MeSH terms
Substances
Associated data
- Actions
- Actions
Grants and funding
LinkOut - more resources
Full Text Sources