Laminin polymerization induces a receptor-cytoskeleton network
- PMID: 10225961
- PMCID: PMC2185083
- DOI: 10.1083/jcb.145.3.619
Laminin polymerization induces a receptor-cytoskeleton network
Abstract
The transition of laminin from a monomeric to a polymerized state is thought to be a crucial step in the development of basement membranes and in the case of skeletal muscle, mutations in laminin can result in severe muscular dystrophies with basement membrane defects. We have evaluated laminin polymer and receptor interactions to determine the requirements for laminin assembly on a cell surface and investigated what cellular responses might be mediated by this transition. We found that on muscle cell surfaces, laminins preferentially polymerize while bound to receptors that included dystroglycan and alpha7beta1 integrin. These receptor interactions are mediated through laminin COOH-terminal domains that are spatially and functionally distinct from NH2-terminal polymer binding sites. This receptor-facilitated self-assembly drives rearrangement of laminin into a cell-associated polygonal network, a process that also requires actin reorganization and tyrosine phosphorylation. As a result, dystroglycan and integrin redistribute into a reciprocal network as do cortical cytoskeleton components vinculin and dystrophin. Cytoskeletal and receptor reorganization is dependent on laminin polymerization and fails in response to receptor occupancy alone (nonpolymerizing laminin). Preferential polymerization of laminin on cell surfaces, and the resulting induction of cortical architecture, is a cooperative process requiring laminin- receptor ligation, receptor-facilitated self-assembly, actin reorganization, and signaling events.
Figures
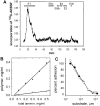
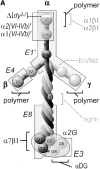
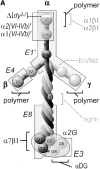
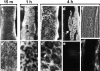
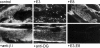
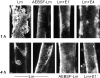
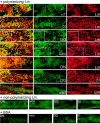
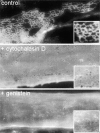
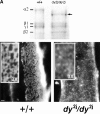
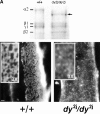
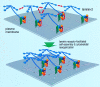
Similar articles
-
Loss of basement membrane, receptor and cytoskeletal lattices in a laminin-deficient muscular dystrophy.J Cell Sci. 2004 Feb 15;117(Pt 5):735-42. doi: 10.1242/jcs.00911. Epub 2004 Jan 20. J Cell Sci. 2004. PMID: 14734655
-
Laminins during muscle development and in muscular dystrophies.Cell Mol Life Sci. 1999 Oct 30;56(5-6):442-60. doi: 10.1007/pl00000616. Cell Mol Life Sci. 1999. PMID: 11212297 Free PMC article. Review.
-
Schwann cell myelination requires integration of laminin activities.J Cell Sci. 2012 Oct 1;125(Pt 19):4609-19. doi: 10.1242/jcs.107995. Epub 2012 Jul 5. J Cell Sci. 2012. PMID: 22767514 Free PMC article.
-
Interactions between dystrophin and the sarcolemma membrane.Soc Gen Physiol Ser. 1997;52:19-29. Soc Gen Physiol Ser. 1997. PMID: 9210217
-
Integrating Activities of Laminins that Drive Basement Membrane Assembly and Function.Curr Top Membr. 2015;76:1-30. doi: 10.1016/bs.ctm.2015.05.001. Epub 2015 Jun 25. Curr Top Membr. 2015. PMID: 26610910 Review.
Cited by
-
Laminin-211 in skeletal muscle function.Cell Adh Migr. 2013 Jan-Feb;7(1):111-21. doi: 10.4161/cam.22618. Epub 2012 Nov 15. Cell Adh Migr. 2013. PMID: 23154401 Free PMC article. Review.
-
The microstructure of laminin-111 compensates for dystroglycan loss in mammary epithelial cells in downstream expression of milk proteins.Biomaterials. 2019 Oct;218:119337. doi: 10.1016/j.biomaterials.2019.119337. Epub 2019 Jul 9. Biomaterials. 2019. PMID: 31325803 Free PMC article.
-
Enzymatically crosslinked gelatin-laminin hydrogels for applications in neuromuscular tissue engineering.Biomater Sci. 2020 Jan 21;8(2):591-606. doi: 10.1039/c9bm01430f. Biomater Sci. 2020. PMID: 31859298 Free PMC article.
-
Phenotypic reversion or death of cancer cells by altering signaling pathways in three-dimensional contexts.J Natl Cancer Inst. 2002 Oct 2;94(19):1494-503. doi: 10.1093/jnci/94.19.1494. J Natl Cancer Inst. 2002. PMID: 12359858 Free PMC article.
-
Developmental and pathogenic mechanisms of basement membrane assembly.Curr Pharm Des. 2009;15(12):1277-94. doi: 10.2174/138161209787846766. Curr Pharm Des. 2009. PMID: 19355968 Free PMC article. Review.
References
-
- Allamand V, Sunada Y, Salih MA, Straub V, Ozo CO, Al-Turaiki MH, Akbar M, Kolo T, Colognato H, Zhang X, Sorokin LM, Yurchenco PD, et al. Mild congenital muscular dystrophy in two patients with an internally deleted laminin alpha2-chain. Hum Mol Genet. 1997;6:747–752. - PubMed
-
- Brown JC, Wiedemann H, Timpl R. Protein binding and cell adhesion properties of two laminin isoforms (AmB1eB2e, AmB1sB2e) from human placenta. J Cell Sci. 1994;107:329–338. - PubMed
-
- Calof AL, Campanero MR, O'Rear JJ, Yurchenco PD, Lander AD. Domain-specific activation of neuronal migration and neurite outgrowth-promoting activities of laminin. Neuron. 1994;13:117–130. - PubMed
Publication types
MeSH terms
Substances
Grants and funding
LinkOut - more resources
Full Text Sources
Other Literature Sources
Research Materials