Minor groove RNA triplex in the crystal structure of a ribosomal frameshifting viral pseudoknot
- PMID: 10074948
- PMCID: PMC7097825
- DOI: 10.1038/6722
Minor groove RNA triplex in the crystal structure of a ribosomal frameshifting viral pseudoknot
Abstract
Many viruses regulate translation of polycistronic mRNA using a -1 ribosomal frameshift induced by an RNA pseudoknot. A pseudoknot has two stems that form a quasi-continuous helix and two connecting loops. A 1.6 A crystal structure of the beet western yellow virus (BWYV) pseudoknot reveals rotation and a bend at the junction of the two stems. A loop base is inserted in the major groove of one stem with quadruple-base interactions. The second loop forms a new minor-groove triplex motif with the other stem, involving 2'-OH and triple-base interactions, as well as sodium ion coordination. Overall, the number of hydrogen bonds stabilizing the tertiary interactions exceeds the number involved in Watson-Crick base pairs. This structure will aid mechanistic analyses of ribosomal frameshifting.
Figures
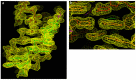
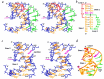
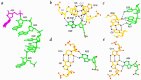
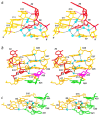
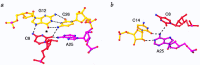
Similar articles
-
Energetics of a strongly pH dependent RNA tertiary structure in a frameshifting pseudoknot.J Mol Biol. 2000 Feb 18;296(2):659-71. doi: 10.1006/jmbi.1999.3464. J Mol Biol. 2000. PMID: 10669615
-
Crystal structure of a luteoviral RNA pseudoknot and model for a minimal ribosomal frameshifting motif.Biochemistry. 2005 Aug 30;44(34):11315-22. doi: 10.1021/bi051061i. Biochemistry. 2005. PMID: 16114868 Free PMC article.
-
A loop 2 cytidine-stem 1 minor groove interaction as a positive determinant for pseudoknot-stimulated -1 ribosomal frameshifting.Proc Natl Acad Sci U S A. 2005 Sep 6;102(36):12694-9. doi: 10.1073/pnas.0506166102. Epub 2005 Aug 25. Proc Natl Acad Sci U S A. 2005. PMID: 16123125 Free PMC article.
-
Structure, stability and function of RNA pseudoknots involved in stimulating ribosomal frameshifting.J Mol Biol. 2000 Apr 28;298(2):167-85. doi: 10.1006/jmbi.2000.3668. J Mol Biol. 2000. PMID: 10764589 Free PMC article. Review.
-
A review on architecture of the gag-pol ribosomal frameshifting RNA in human immunodeficiency virus: a variability survey of virus genotypes.J Biomol Struct Dyn. 2017 Jun;35(8):1629-1653. doi: 10.1080/07391102.2016.1194231. Epub 2016 Aug 2. J Biomol Struct Dyn. 2017. PMID: 27485859 Review.
Cited by
-
Viral RNA pseudoknots: versatile motifs in gene expression and replication.Nat Rev Microbiol. 2007 Aug;5(8):598-610. doi: 10.1038/nrmicro1704. Nat Rev Microbiol. 2007. PMID: 17632571 Free PMC article. Review.
-
Programmed ribosomal frameshifting: much ado about knotting!Proc Natl Acad Sci U S A. 1999 Dec 7;96(25):14177-9. doi: 10.1073/pnas.96.25.14177. Proc Natl Acad Sci U S A. 1999. PMID: 10588670 Free PMC article. No abstract available.
-
NCIR: a database of non-canonical interactions in known RNA structures.Nucleic Acids Res. 2002 Jan 1;30(1):395-7. doi: 10.1093/nar/30.1.395. Nucleic Acids Res. 2002. PMID: 11752347 Free PMC article.
-
Footprinting analysis of BWYV pseudoknot-ribosome complexes.RNA. 2009 Sep;15(9):1775-86. doi: 10.1261/rna.1385409. Epub 2009 Jul 22. RNA. 2009. PMID: 19625386 Free PMC article.
-
The global structures of a wild-type and poorly functional plant luteoviral mRNA pseudoknot are essentially identical.RNA. 2006 Nov;12(11):1959-69. doi: 10.1261/rna.199006. Epub 2006 Sep 25. RNA. 2006. PMID: 17000902 Free PMC article.
References
Publication types
MeSH terms
Substances
Associated data
- Actions
LinkOut - more resources
Full Text Sources