Abstract
Acetylcholinesterases (AChEs) are characterized by a high net negative charge and by an uneven surface charge distribution, giving rise to a negative electrostatic potential extending over most of the molecular surface. To evaluate the contribution of these electrostatic properties to the catalytic efficiency, 20 single- and multiple-site mutants of human AChE were generated by replacing up to seven acidic residues, vicinal to the rim of the active-center gorge (Glu84, Glu285, Glu292, Asp349, Glu358, Glu389 and Asp390), by neutral amino acids. Progressive simulated replacement of these charged residues results in a gradual decrease of the negative electrostatic potential which is essentially eliminated by neutralizing six or seven charges. In marked contrast to the shrinking of the electrostatic potential, the corresponding mutations had no significant effect on the apparent bimolecular rate constants of hydrolysis for charged and non-charged substrates, or on the Ki value for a charged active center inhibitor. Moreover, the kcat values for all 20 mutants are essentially identical to that of the wild type enzyme, and the apparent bimolecular rate constants show a moderate dependence on the ionic strength, which is invariant for all the enzymes examined. These findings suggest that the surface electrostatic properties of AChE do not contribute to the catalytic rate, that this rate is probably not diffusion-controlled and that long-range electrostatic interactions play no role in stabilization of the transition states of the catalytic process.
Full text
PDF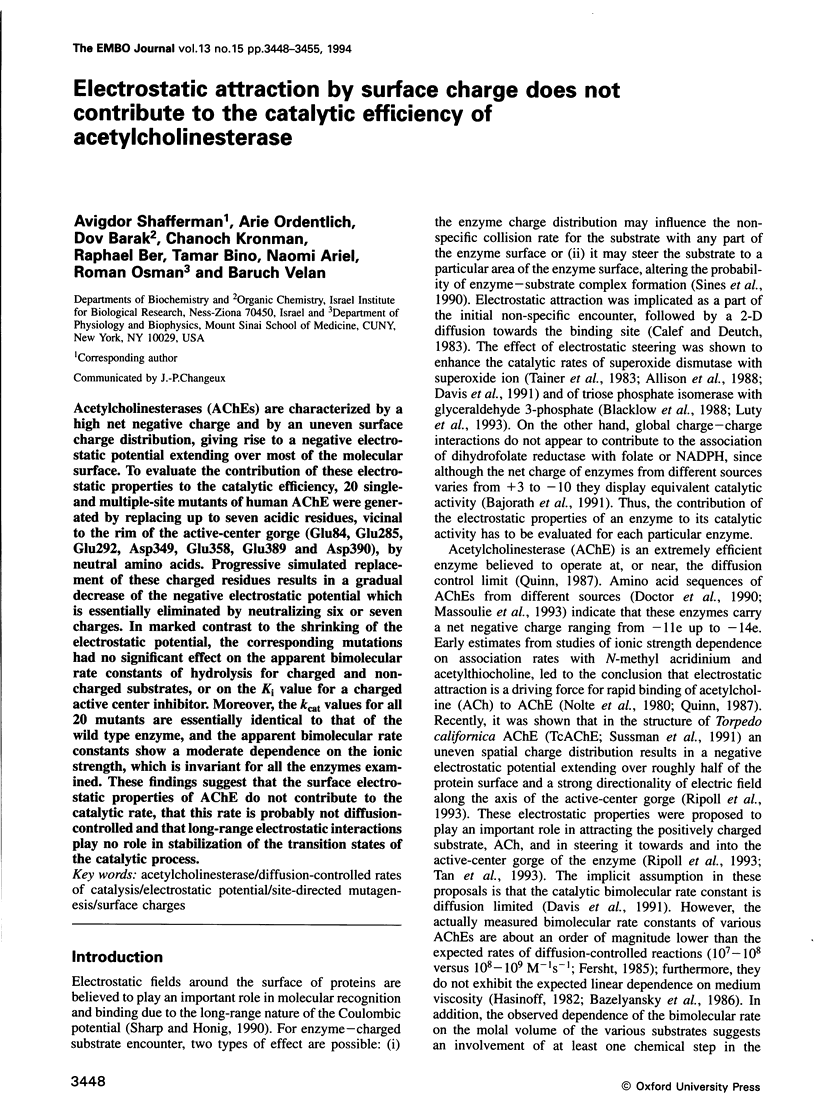
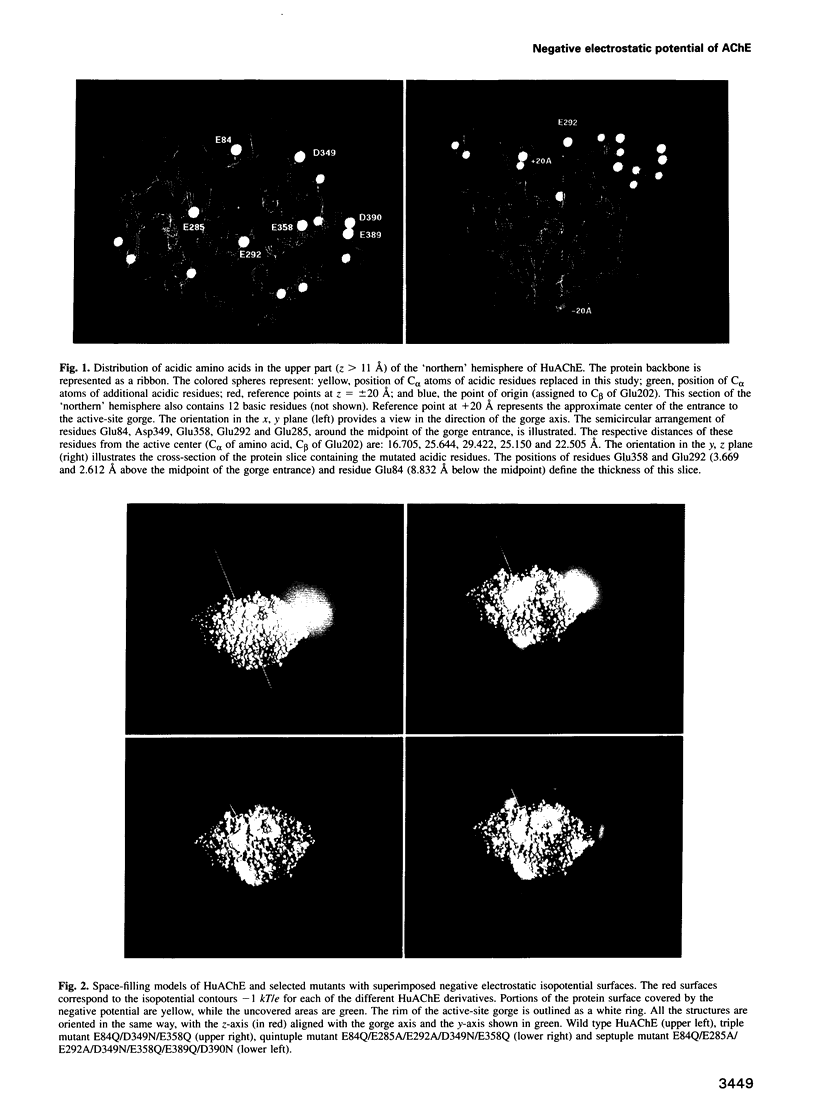
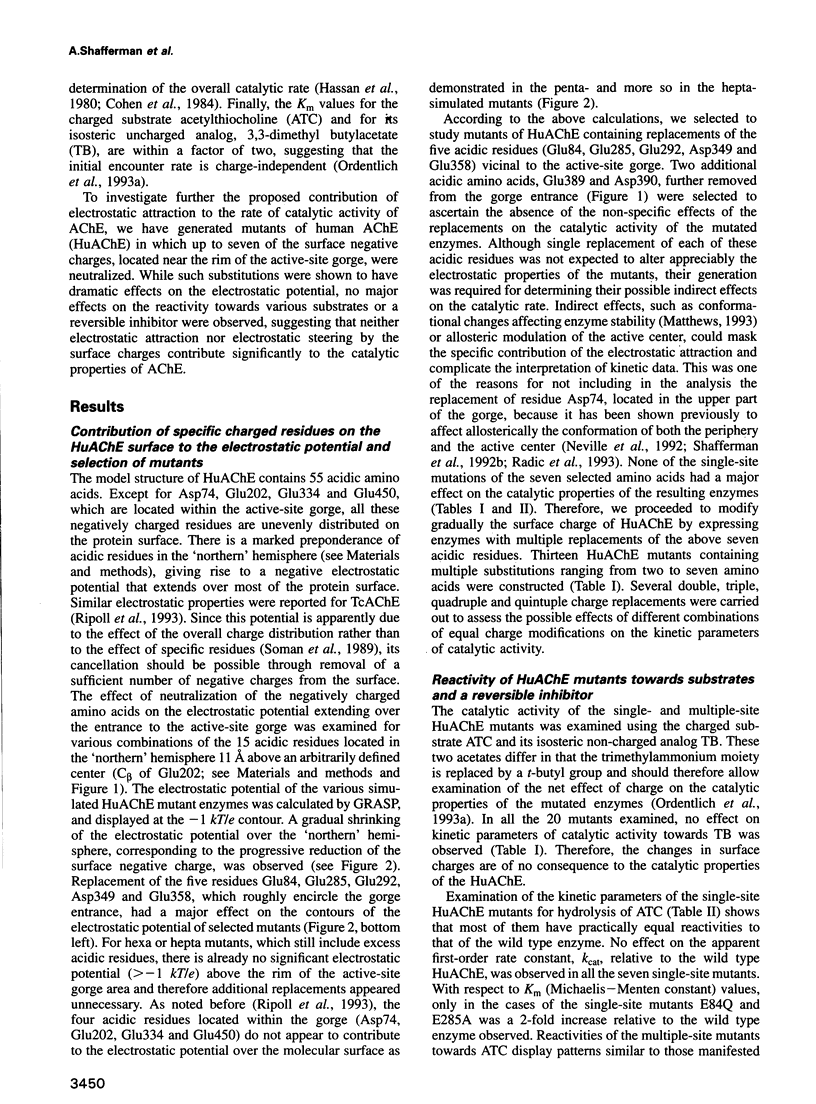
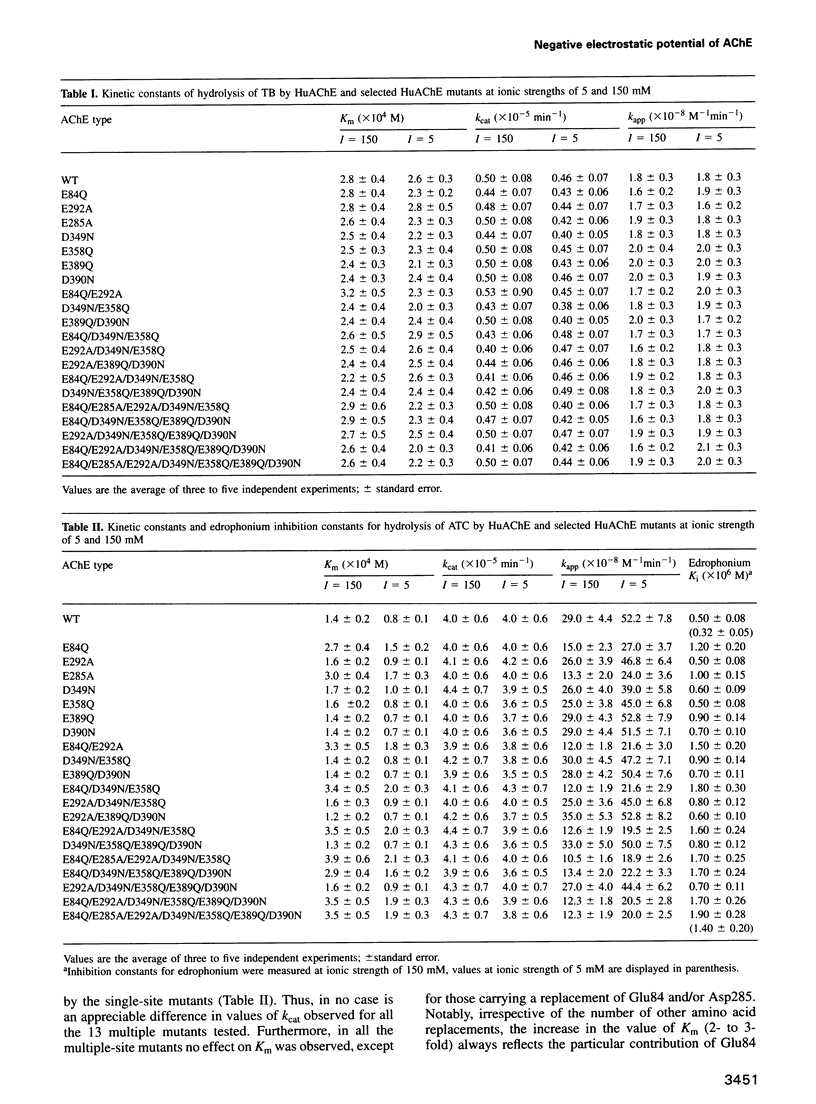
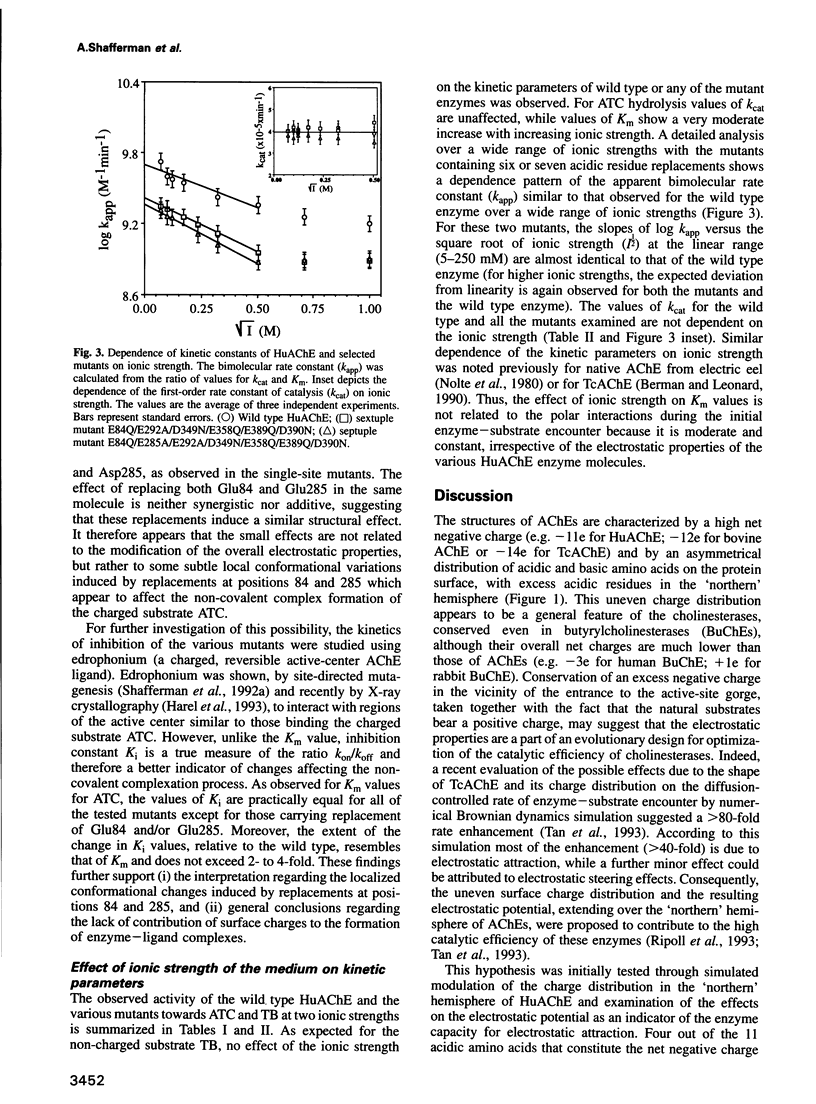
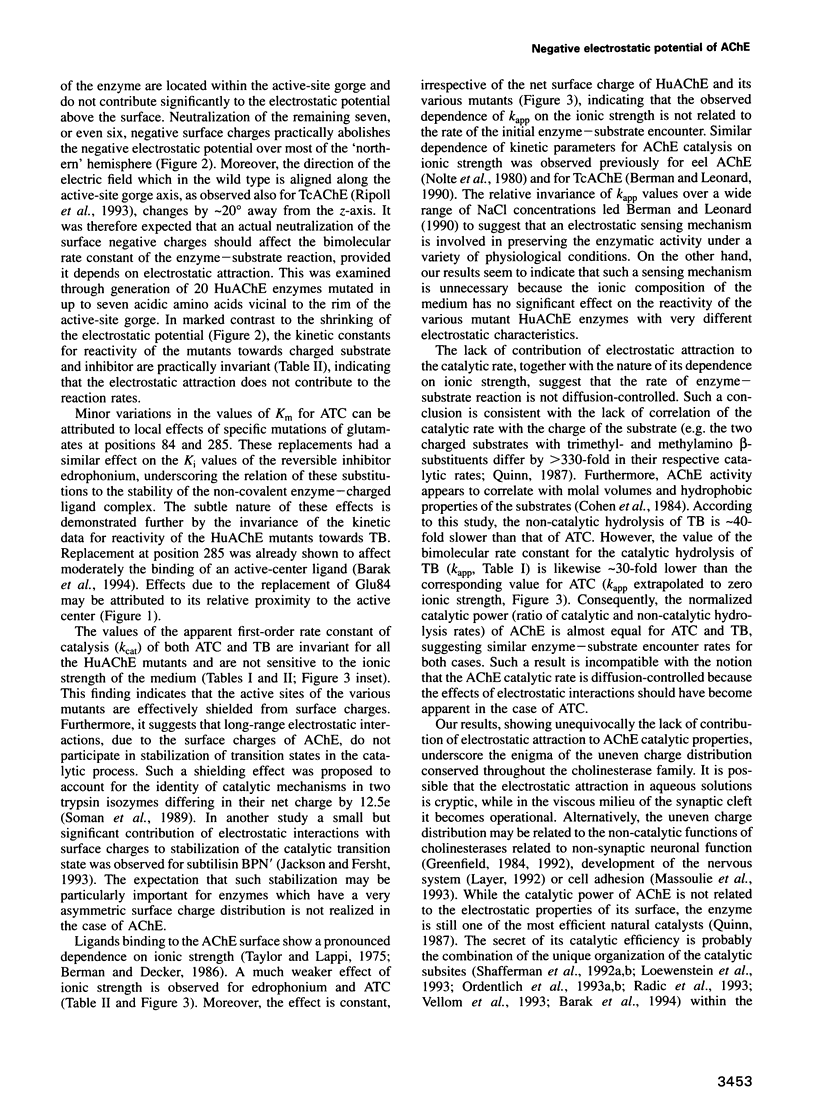
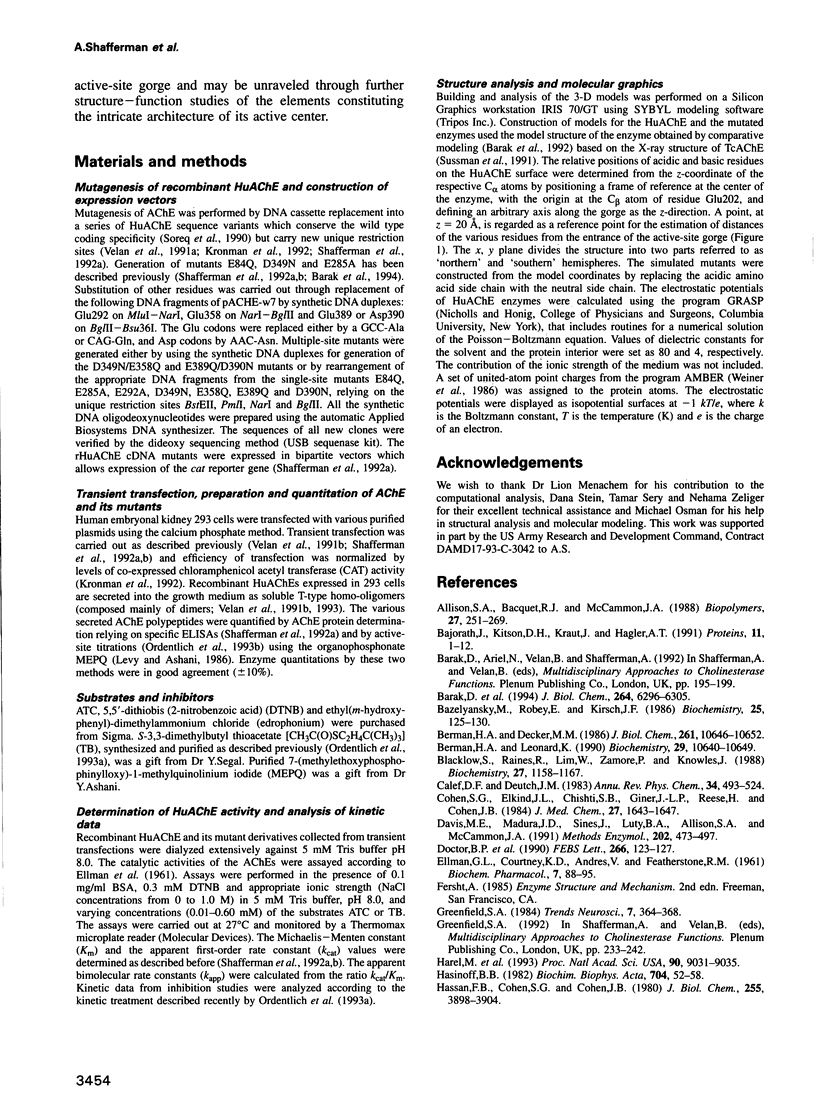
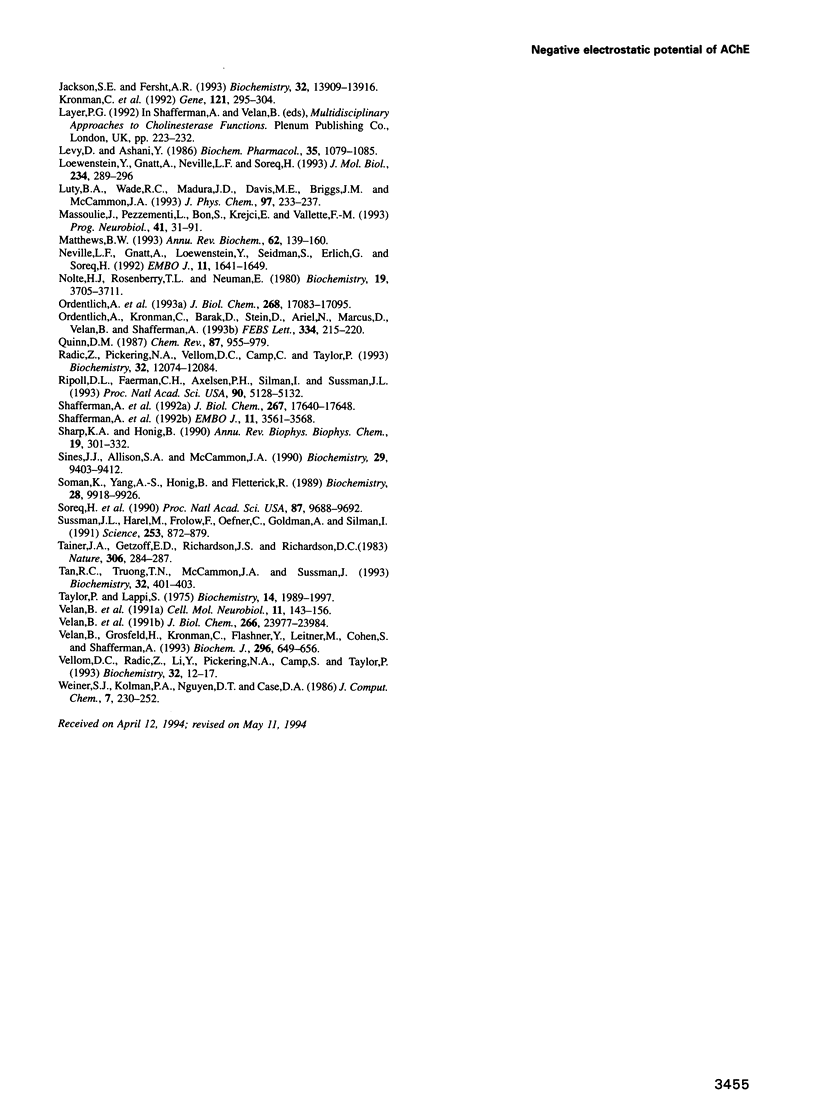
Images in this article
Selected References
These references are in PubMed. This may not be the complete list of references from this article.
- Allison S. A., Bacquet R. J., McCammon J. A. Simulation of the diffusion-controlled reaction between superoxide and superoxide dismutase. II. Detailed models. Biopolymers. 1988 Feb;27(2):251–269. doi: 10.1002/bip.360270207. [DOI] [PubMed] [Google Scholar]
- Bajorath J., Kitson D. H., Kraut J., Hagler A. T. The electrostatic potential of Escherichia coli dihydrofolate reductase. Proteins. 1991;11(1):1–12. doi: 10.1002/prot.340110102. [DOI] [PubMed] [Google Scholar]
- Barak D., Kronman C., Ordentlich A., Ariel N., Bromberg A., Marcus D., Lazar A., Velan B., Shafferman A. Acetylcholinesterase peripheral anionic site degeneracy conferred by amino acid arrays sharing a common core. J Biol Chem. 1994 Mar 4;269(9):6296–6305. [PubMed] [Google Scholar]
- Bazelyansky M., Robey E., Kirsch J. F. Fractional diffusion-limited component of reactions catalyzed by acetylcholinesterase. Biochemistry. 1986 Jan 14;25(1):125–130. doi: 10.1021/bi00349a019. [DOI] [PubMed] [Google Scholar]
- Berman H. A., Decker M. M. Kinetic, equilibrium, and spectroscopic studies on dealkylation ("aging") of alkyl organophosphonyl acetylcholinesterase. Electrostatic control of enzyme topography. J Biol Chem. 1986 Aug 15;261(23):10646–10652. [PubMed] [Google Scholar]
- Berman H. A., Leonard K. Ligand exclusion on acetylcholinesterase. Biochemistry. 1990 Nov 27;29(47):10640–10649. doi: 10.1021/bi00499a010. [DOI] [PubMed] [Google Scholar]
- Blacklow S. C., Raines R. T., Lim W. A., Zamore P. D., Knowles J. R. Triosephosphate isomerase catalysis is diffusion controlled. Appendix: Analysis of triose phosphate equilibria in aqueous solution by 31P NMR. Biochemistry. 1988 Feb 23;27(4):1158–1167. doi: 10.1021/bi00404a013. [DOI] [PubMed] [Google Scholar]
- Cohen S. G., Elkind J. L., Chishti S. B., Giner J. L., Reese H., Cohen J. B. Effects of volume and surface property in hydrolysis by acetylcholinesterase. The trimethyl site. J Med Chem. 1984 Dec;27(12):1643–1647. doi: 10.1021/jm00378a020. [DOI] [PubMed] [Google Scholar]
- Davis M. E., Madura J. D., Sines J., Luty B. A., Allison S. A., McCammon J. A. Diffusion-controlled enzymatic reactions. Methods Enzymol. 1991;202:473–497. doi: 10.1016/0076-6879(91)02024-4. [DOI] [PubMed] [Google Scholar]
- Doctor B. P., Chapman T. C., Christner C. E., Deal C. D., De La Hoz D. M., Gentry M. K., Ogert R. A., Rush R. S., Smyth K. K., Wolfe A. D. Complete amino acid sequence of fetal bovine serum acetylcholinesterase and its comparison in various regions with other cholinesterases. FEBS Lett. 1990 Jun 18;266(1-2):123–127. doi: 10.1016/0014-5793(90)81522-p. [DOI] [PubMed] [Google Scholar]
- ELLMAN G. L., COURTNEY K. D., ANDRES V., Jr, FEATHER-STONE R. M. A new and rapid colorimetric determination of acetylcholinesterase activity. Biochem Pharmacol. 1961 Jul;7:88–95. doi: 10.1016/0006-2952(61)90145-9. [DOI] [PubMed] [Google Scholar]
- Harel M., Schalk I., Ehret-Sabatier L., Bouet F., Goeldner M., Hirth C., Axelsen P. H., Silman I., Sussman J. L. Quaternary ligand binding to aromatic residues in the active-site gorge of acetylcholinesterase. Proc Natl Acad Sci U S A. 1993 Oct 1;90(19):9031–9035. doi: 10.1073/pnas.90.19.9031. [DOI] [PMC free article] [PubMed] [Google Scholar]
- Hasan F. B., Cohen S. G., Cohen J. B. Hydrolysis by acetylcholinesterase. Apparent molal volumes and trimethyl and methyl subsites. J Biol Chem. 1980 May 10;255(9):3898–3904. [PubMed] [Google Scholar]
- Hasinoff B. B. Kinetics of acetylthiocholine binding to electric eel acetylcholinesterase in glycerol/water solvents of increased viscosity. Evidence for a diffusion-controlled reaction. Biochim Biophys Acta. 1982 May 21;704(1):52–58. doi: 10.1016/0167-4838(82)90131-5. [DOI] [PubMed] [Google Scholar]
- Jackson S. E., Fersht A. R. Contribution of long-range electrostatic interactions to the stabilization of the catalytic transition state of the serine protease subtilisin BPN'. Biochemistry. 1993 Dec 21;32(50):13909–13916. doi: 10.1021/bi00213a021. [DOI] [PubMed] [Google Scholar]
- Kronman C., Velan B., Gozes Y., Leitner M., Flashner Y., Lazar A., Marcus D., Sery T., Papier Y., Grosfeld H. Production and secretion of high levels of recombinant human acetylcholinesterase in cultured cell lines: microheterogeneity of the catalytic subunit. Gene. 1992 Nov 16;121(2):295–304. doi: 10.1016/0378-1119(92)90134-b. [DOI] [PubMed] [Google Scholar]
- Levy D., Ashani Y. Synthesis and in vitro properties of a powerful quaternary methylphosphonate inhibitor of acetylcholinesterase. A new marker in blood-brain barrier research. Biochem Pharmacol. 1986 Apr 1;35(7):1079–1085. doi: 10.1016/0006-2952(86)90142-5. [DOI] [PubMed] [Google Scholar]
- Loewenstein Y., Gnatt A., Neville L. F., Soreq H. Chimeric human cholinesterase. Identification of interaction sites responsible for recognition of acetyl- or butyrylcholinesterase-specific ligands. J Mol Biol. 1993 Nov 20;234(2):289–296. doi: 10.1006/jmbi.1993.1584. [DOI] [PubMed] [Google Scholar]
- Massoulié J., Pezzementi L., Bon S., Krejci E., Vallette F. M. Molecular and cellular biology of cholinesterases. Prog Neurobiol. 1993 Jul;41(1):31–91. doi: 10.1016/0301-0082(93)90040-y. [DOI] [PubMed] [Google Scholar]
- Matthews B. W. Structural and genetic analysis of protein stability. Annu Rev Biochem. 1993;62:139–160. doi: 10.1146/annurev.bi.62.070193.001035. [DOI] [PubMed] [Google Scholar]
- Neville L. F., Gnatt A., Loewenstein Y., Seidman S., Ehrlich G., Soreq H. Intramolecular relationships in cholinesterases revealed by oocyte expression of site-directed and natural variants of human BCHE. EMBO J. 1992 Apr;11(4):1641–1649. doi: 10.1002/j.1460-2075.1992.tb05210.x. [DOI] [PMC free article] [PubMed] [Google Scholar]
- Nolte H. J., Rosenberry T. L., Neumann E. Effective charge on acetylcholinesterase active sites determined from the ionic strength dependence of association rate constants with cationic ligands. Biochemistry. 1980 Aug 5;19(16):3705–3711. doi: 10.1021/bi00557a011. [DOI] [PubMed] [Google Scholar]
- Ordentlich A., Barak D., Kronman C., Flashner Y., Leitner M., Segall Y., Ariel N., Cohen S., Velan B., Shafferman A. Dissection of the human acetylcholinesterase active center determinants of substrate specificity. Identification of residues constituting the anionic site, the hydrophobic site, and the acyl pocket. J Biol Chem. 1993 Aug 15;268(23):17083–17095. [PubMed] [Google Scholar]
- Ordentlich A., Kronman C., Barak D., Stein D., Ariel N., Marcus D., Velan B., Shafferman A. Engineering resistance to 'aging' of phosphylated human acetylcholinesterase. Role of hydrogen bond network in the active center. FEBS Lett. 1993 Nov 15;334(2):215–220. doi: 10.1016/0014-5793(93)81714-b. [DOI] [PubMed] [Google Scholar]
- Radić Z., Pickering N. A., Vellom D. C., Camp S., Taylor P. Three distinct domains in the cholinesterase molecule confer selectivity for acetyl- and butyrylcholinesterase inhibitors. Biochemistry. 1993 Nov 16;32(45):12074–12084. doi: 10.1021/bi00096a018. [DOI] [PubMed] [Google Scholar]
- Ripoll D. R., Faerman C. H., Axelsen P. H., Silman I., Sussman J. L. An electrostatic mechanism for substrate guidance down the aromatic gorge of acetylcholinesterase. Proc Natl Acad Sci U S A. 1993 Jun 1;90(11):5128–5132. doi: 10.1073/pnas.90.11.5128. [DOI] [PMC free article] [PubMed] [Google Scholar]
- Shafferman A., Kronman C., Flashner Y., Leitner M., Grosfeld H., Ordentlich A., Gozes Y., Cohen S., Ariel N., Barak D. Mutagenesis of human acetylcholinesterase. Identification of residues involved in catalytic activity and in polypeptide folding. J Biol Chem. 1992 Sep 5;267(25):17640–17648. [PubMed] [Google Scholar]
- Shafferman A., Velan B., Ordentlich A., Kronman C., Grosfeld H., Leitner M., Flashner Y., Cohen S., Barak D., Ariel N. Substrate inhibition of acetylcholinesterase: residues affecting signal transduction from the surface to the catalytic center. EMBO J. 1992 Oct;11(10):3561–3568. doi: 10.1002/j.1460-2075.1992.tb05439.x. [DOI] [PMC free article] [PubMed] [Google Scholar]
- Sharp K. A., Honig B. Electrostatic interactions in macromolecules: theory and applications. Annu Rev Biophys Biophys Chem. 1990;19:301–332. doi: 10.1146/annurev.bb.19.060190.001505. [DOI] [PubMed] [Google Scholar]
- Sines J. J., Allison S. A., McCammon J. A. Point charge distributions and electrostatic steering in enzyme/substrate encounter: Brownian dynamics of modified copper/zinc superoxide dismutases. Biochemistry. 1990 Oct 9;29(40):9403–9412. doi: 10.1021/bi00492a014. [DOI] [PubMed] [Google Scholar]
- Soman K., Yang A. S., Honig B., Fletterick R. Electrical potentials in trypsin isozymes. Biochemistry. 1989 Dec 26;28(26):9918–9926. doi: 10.1021/bi00452a007. [DOI] [PubMed] [Google Scholar]
- Soreq H., Ben-Aziz R., Prody C. A., Seidman S., Gnatt A., Neville L., Lieman-Hurwitz J., Lev-Lehman E., Ginzberg D., Lipidot-Lifson Y. Molecular cloning and construction of the coding region for human acetylcholinesterase reveals a G + C-rich attenuating structure. Proc Natl Acad Sci U S A. 1990 Dec;87(24):9688–9692. doi: 10.1073/pnas.87.24.9688. [DOI] [PMC free article] [PubMed] [Google Scholar]
- Sussman J. L., Harel M., Frolow F., Oefner C., Goldman A., Toker L., Silman I. Atomic structure of acetylcholinesterase from Torpedo californica: a prototypic acetylcholine-binding protein. Science. 1991 Aug 23;253(5022):872–879. doi: 10.1126/science.1678899. [DOI] [PubMed] [Google Scholar]
- Tainer J. A., Getzoff E. D., Richardson J. S., Richardson D. C. Structure and mechanism of copper, zinc superoxide dismutase. Nature. 1983 Nov 17;306(5940):284–287. doi: 10.1038/306284a0. [DOI] [PubMed] [Google Scholar]
- Tan R. C., Truong T. N., McCammon J. A., Sussman J. L. Acetylcholinesterase: electrostatic steering increases the rate of ligand binding. Biochemistry. 1993 Jan 19;32(2):401–403. doi: 10.1021/bi00053a003. [DOI] [PubMed] [Google Scholar]
- Taylor P., Lappi S. Interaction of fluorescence probes with acetylcholinesterase. The site and specificity of propidium binding. Biochemistry. 1975 May 6;14(9):1989–1997. doi: 10.1021/bi00680a029. [DOI] [PubMed] [Google Scholar]
- Velan B., Grosfeld H., Kronman C., Leitner M., Gozes Y., Lazar A., Flashner Y., Marcus D., Cohen S., Shafferman A. The effect of elimination of intersubunit disulfide bonds on the activity, assembly, and secretion of recombinant human acetylcholinesterase. Expression of acetylcholinesterase Cys-580----Ala mutant. J Biol Chem. 1991 Dec 15;266(35):23977–23984. [PubMed] [Google Scholar]
- Velan B., Kronman C., Grosfeld H., Leitner M., Gozes Y., Flashner Y., Sery T., Cohen S., Ben-Aziz R., Seidman S. Recombinant human acetylcholinesterase is secreted from transiently transfected 293 cells as a soluble globular enzyme. Cell Mol Neurobiol. 1991 Feb;11(1):143–156. doi: 10.1007/BF00712806. [DOI] [PMC free article] [PubMed] [Google Scholar]
- Velan B., Kronman C., Ordentlich A., Flashner Y., Leitner M., Cohen S., Shafferman A. N-glycosylation of human acetylcholinesterase: effects on activity, stability and biosynthesis. Biochem J. 1993 Dec 15;296(Pt 3):649–656. doi: 10.1042/bj2960649. [DOI] [PMC free article] [PubMed] [Google Scholar]
- Vellom D. C., Radić Z., Li Y., Pickering N. A., Camp S., Taylor P. Amino acid residues controlling acetylcholinesterase and butyrylcholinesterase specificity. Biochemistry. 1993 Jan 12;32(1):12–17. doi: 10.1021/bi00052a003. [DOI] [PubMed] [Google Scholar]