Abstract
To probe protonation dynamics inside the fully open alpha-toxin ion channel, we measured the pH-dependent fluctuations in its current. In the presence of 1 M NaCl dissolved in H2O and positive applied potentials (from the side of protein addition), the low frequency noise exhibited a single well defined peak between pH 4.5 and 7.5. A simple model in which the current is assumed to change by equal amounts upon the reversible protonation of each of N identical ionizable residues inside the channel describes the data well. These results, and the frequency dependence of the spectral density at higher frequencies, allow us to evaluate the effective pK = 5.5, as well as the rate constants for the reversible protonation reactions: kon = 8 x 10(9) M-1 s-1 and koff = 2.5 x 10(4) s-1. The estimate of kon is only slightly less than the diffusion-limited values measured by others for protonation reactions for free carboxyl or imidazole residues. Substitution of H2O by D2O caused a 3.8-fold decrease in the dissociation rate constant and shifted the pK to 6.0. The decrease in the ionization rate constants caused by H2O/D2O substitution permitted the reliable measurement of the characteristic relaxation time over a wide range of D+ concentrations and voltages. The dependence of the relaxation time on D+ concentration strongly supports the first order reaction model. The voltage dependence of the low frequency spectral density suggests that the protonation dynamics are virtually insensitive to the applied potential while the rate-limiting barriers for NaCl transport are voltage dependent. The number of ionizable residues deduced from experiments in H2O (N = 4.2) and D2O (N = 4.1) is in good agreement.
Full text
PDF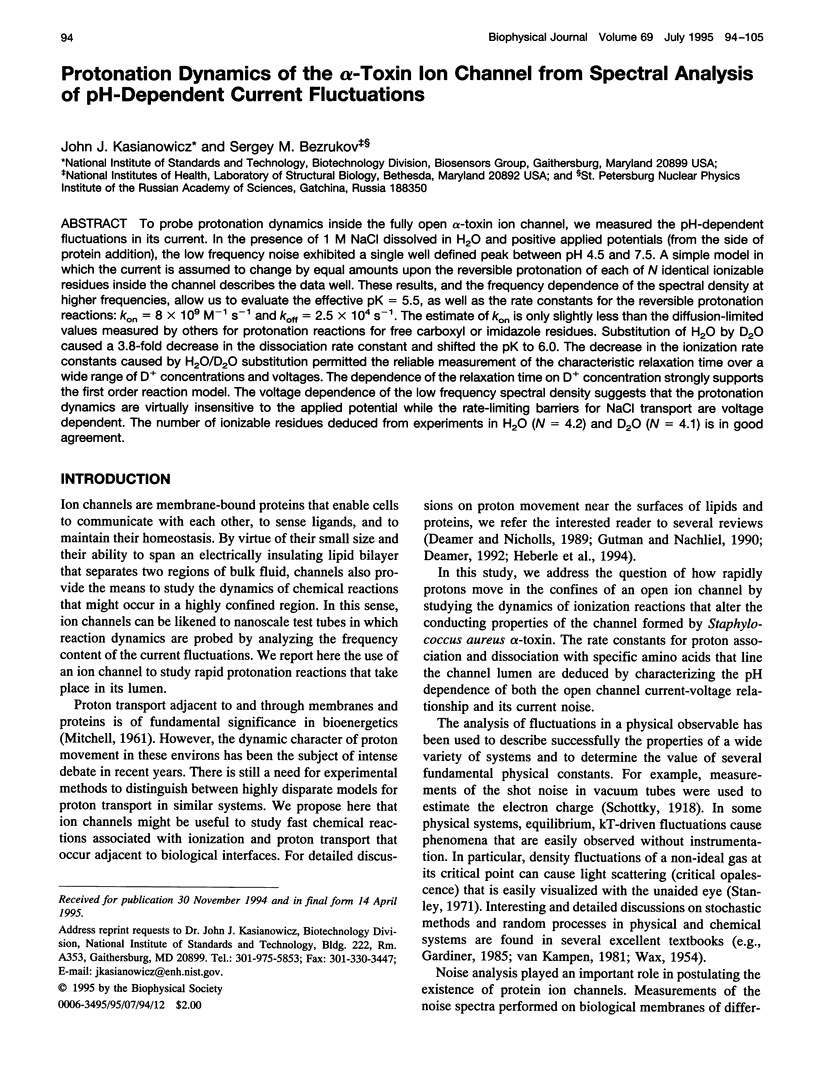
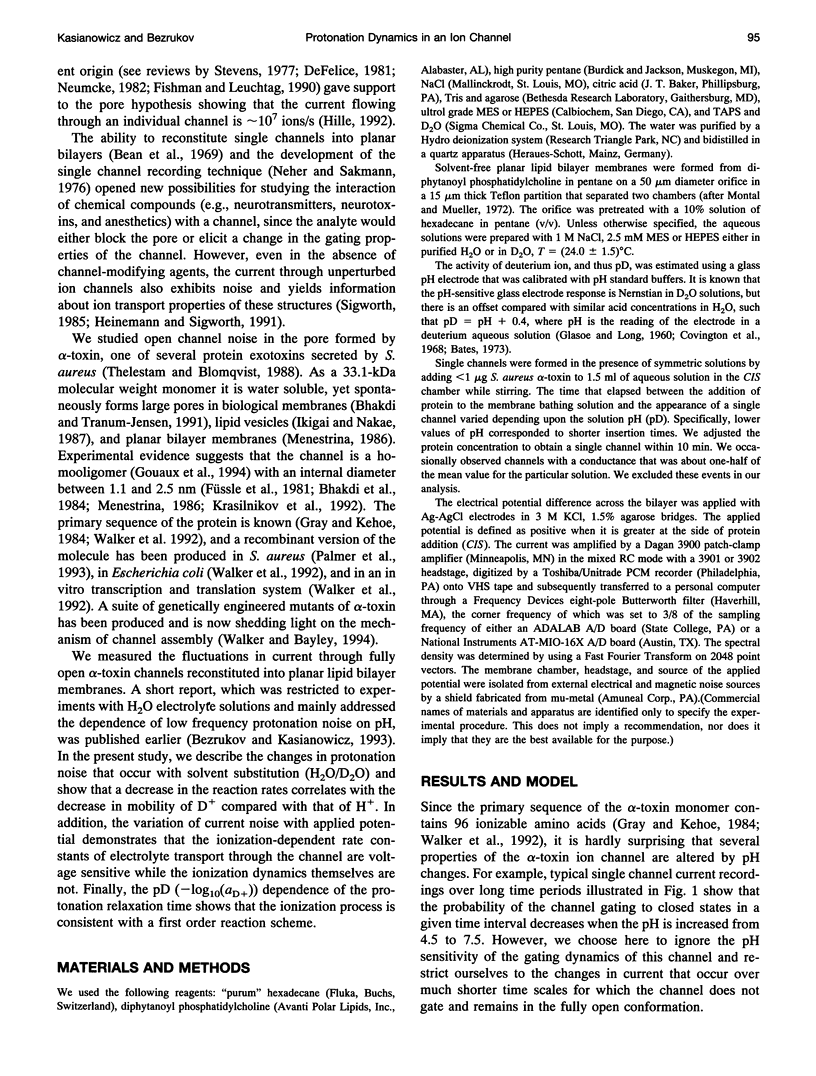
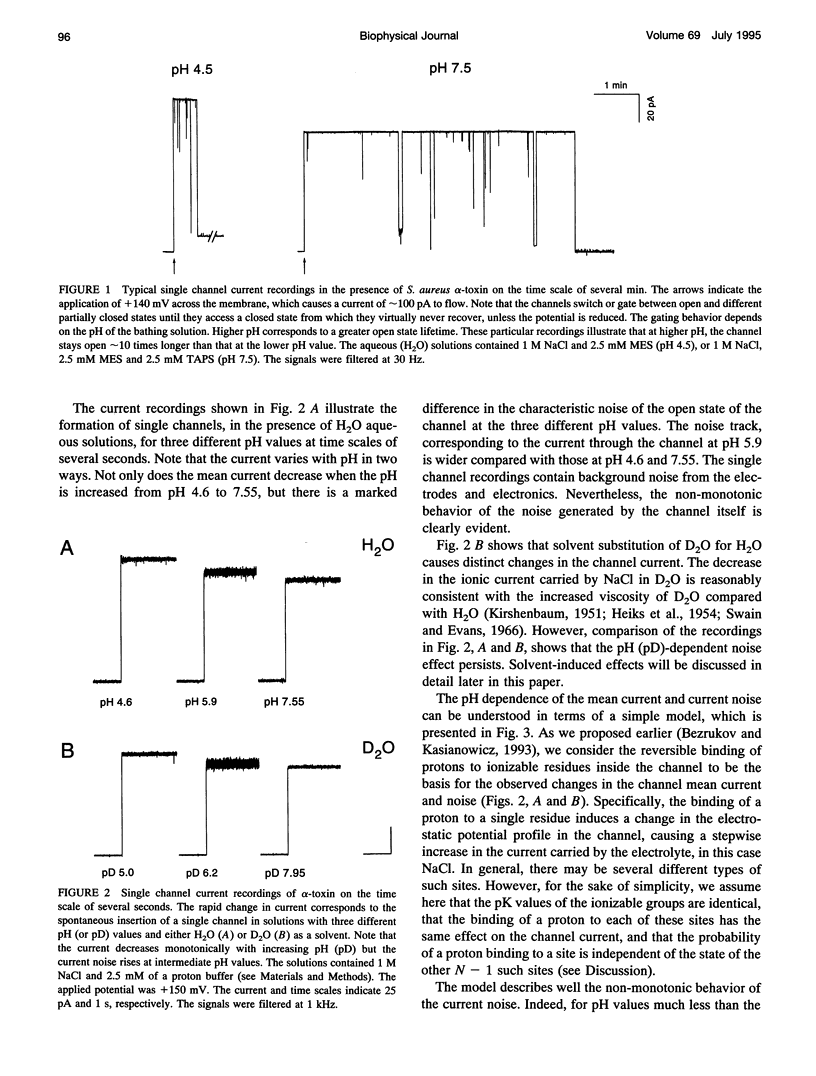
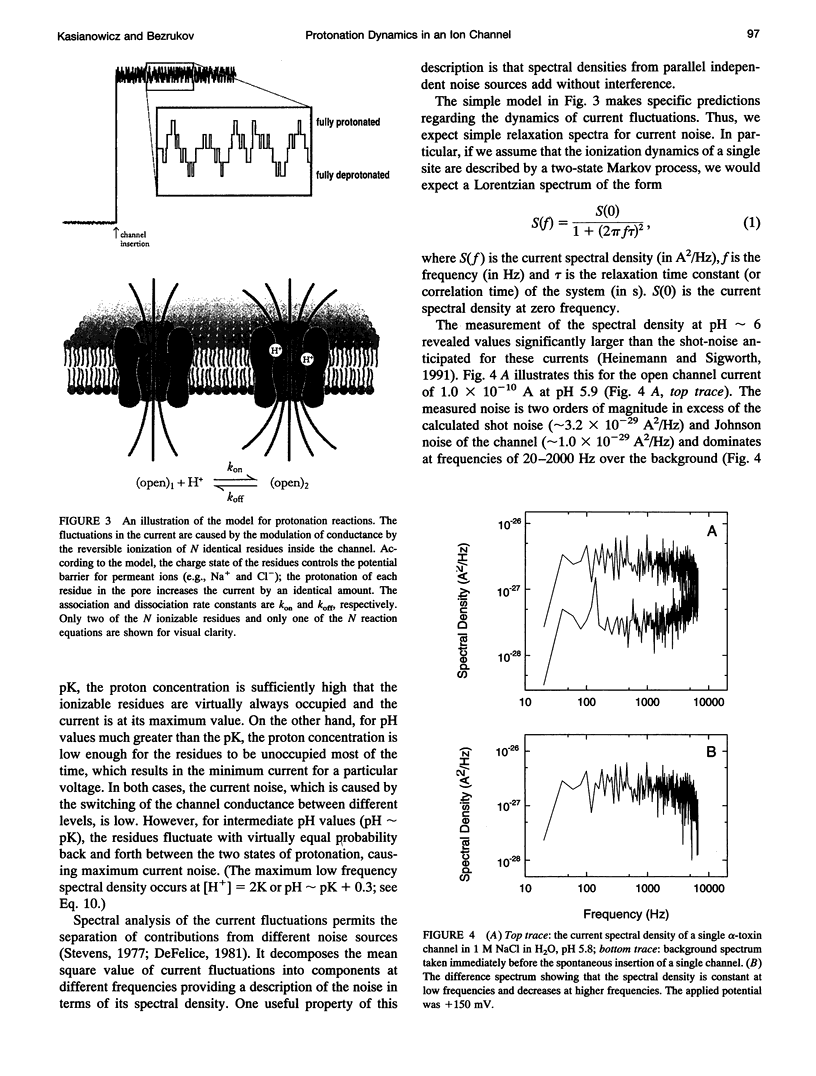
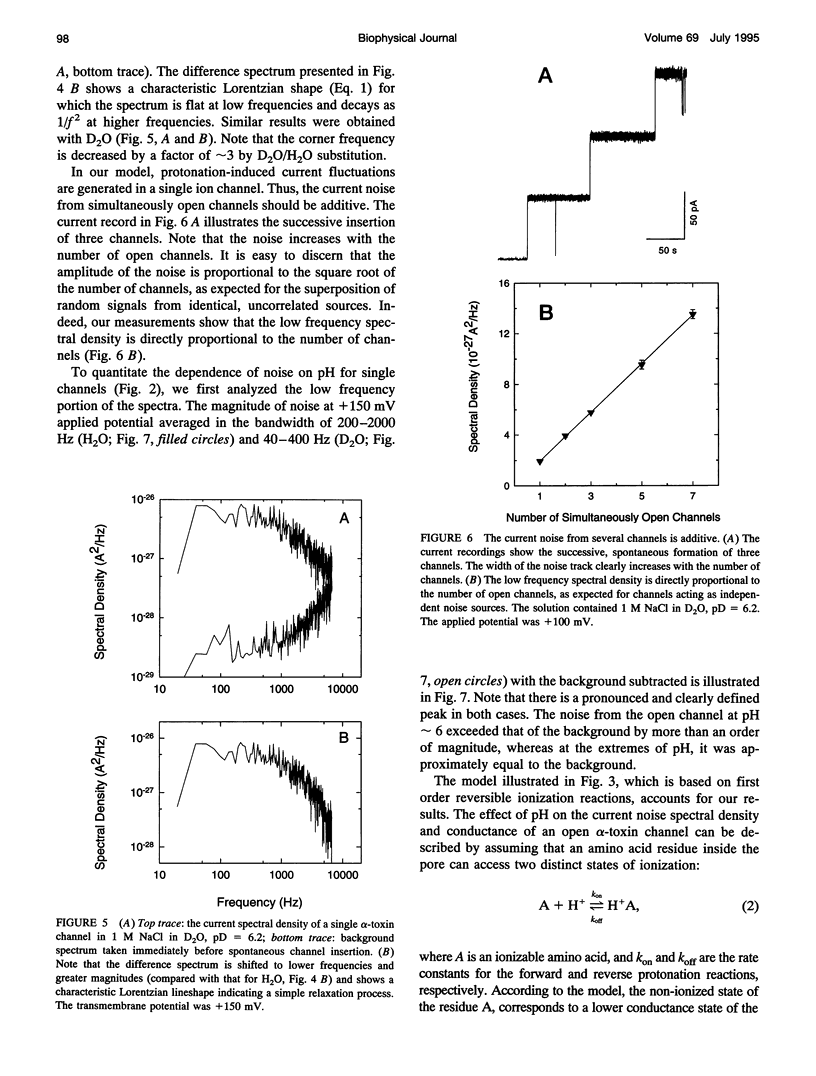
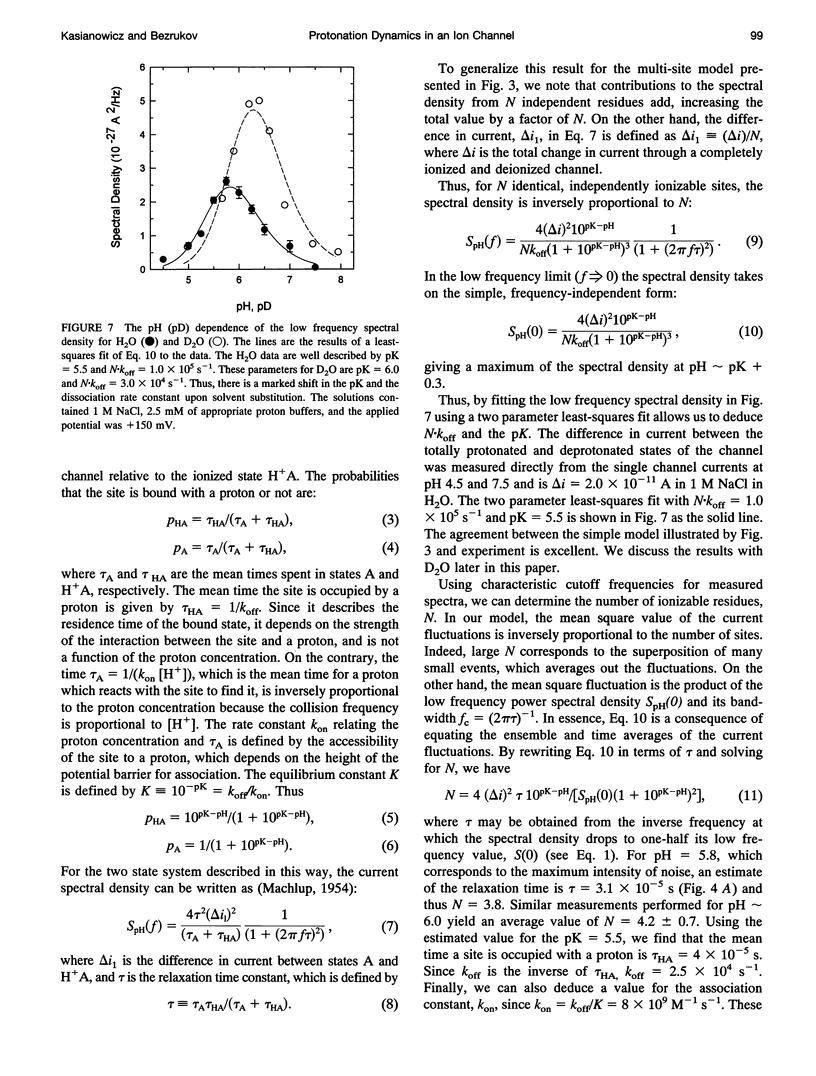
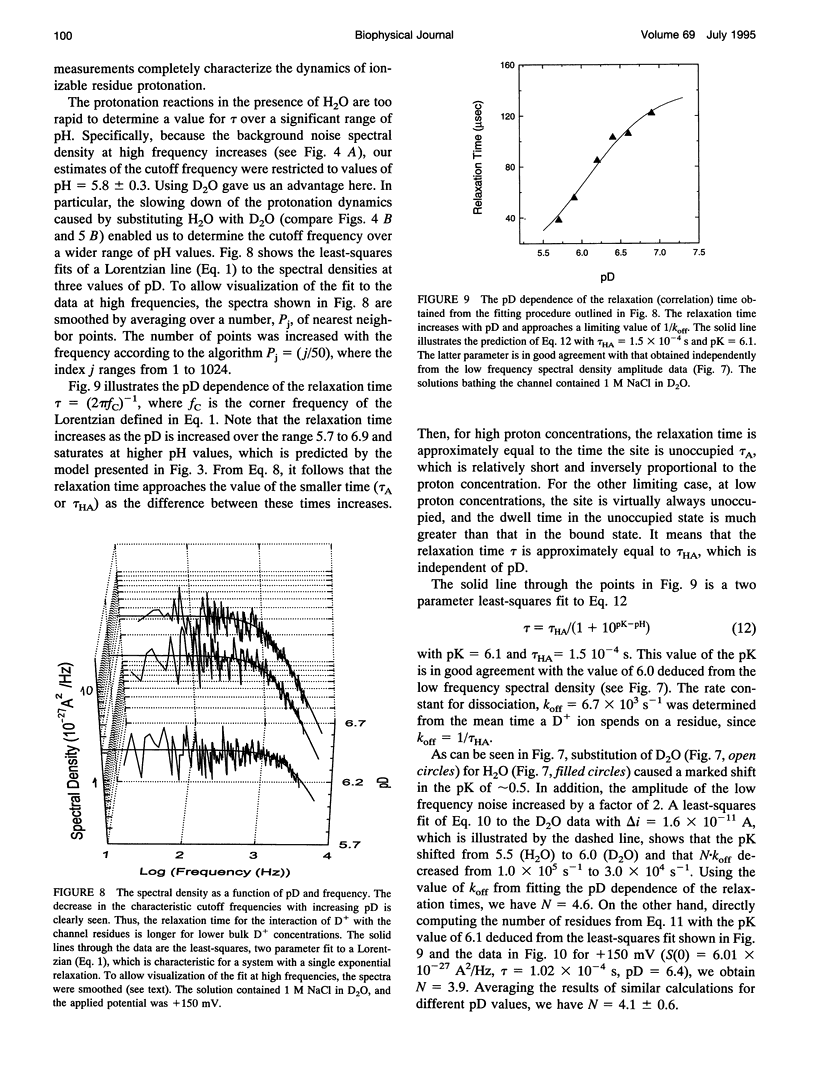
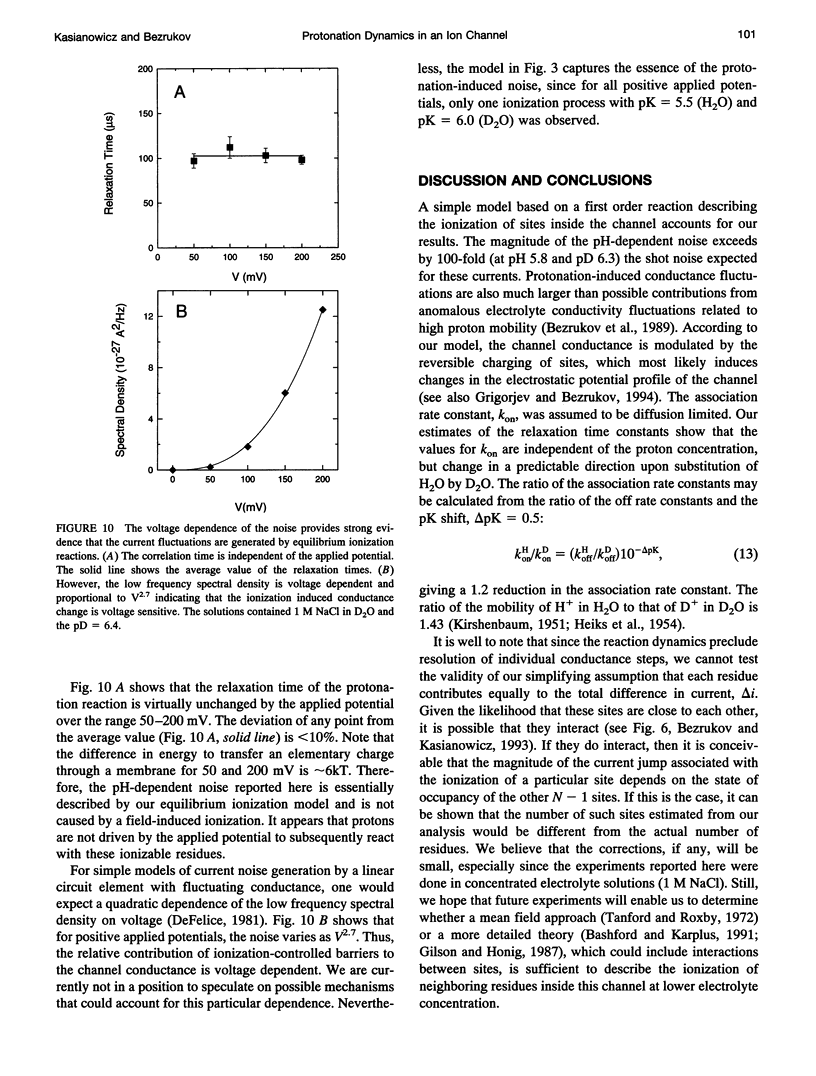
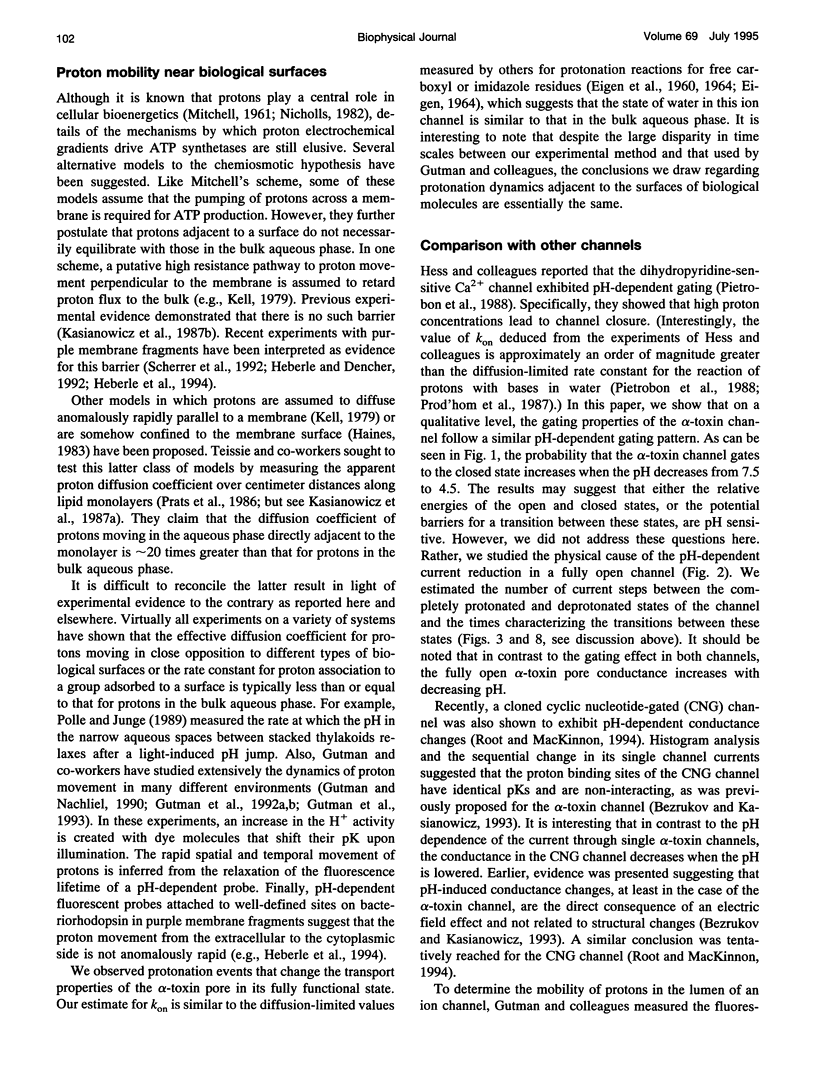
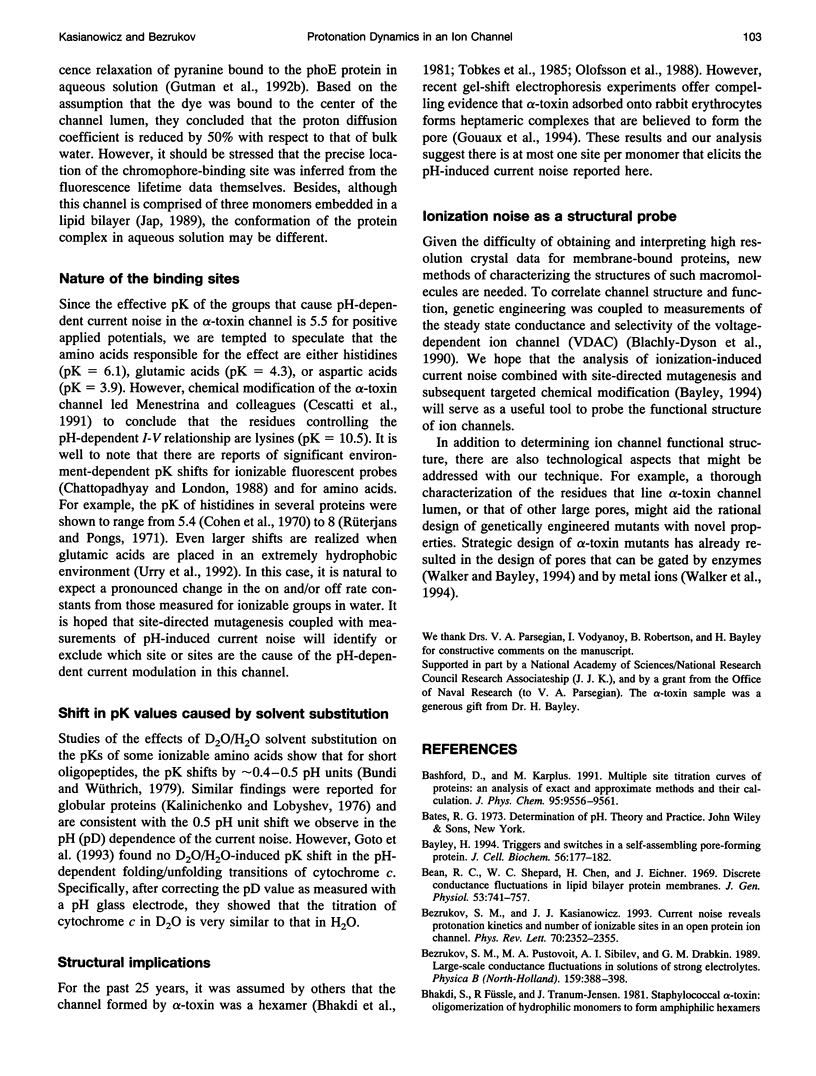
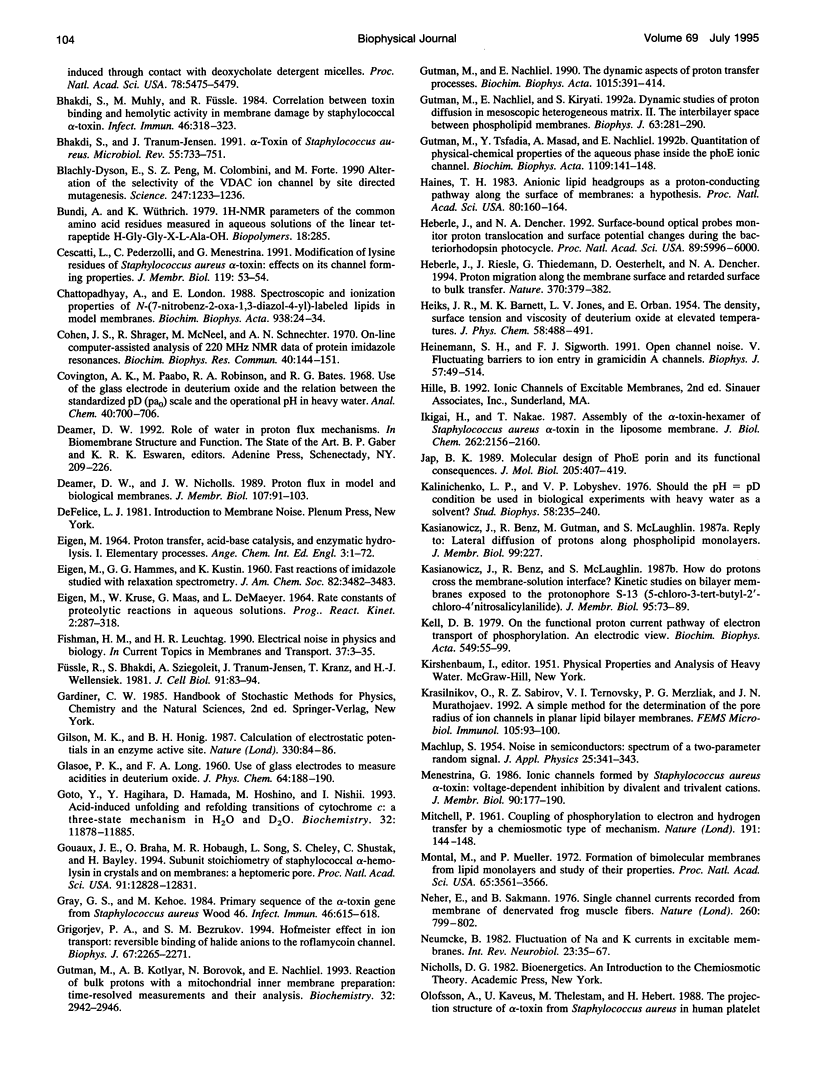
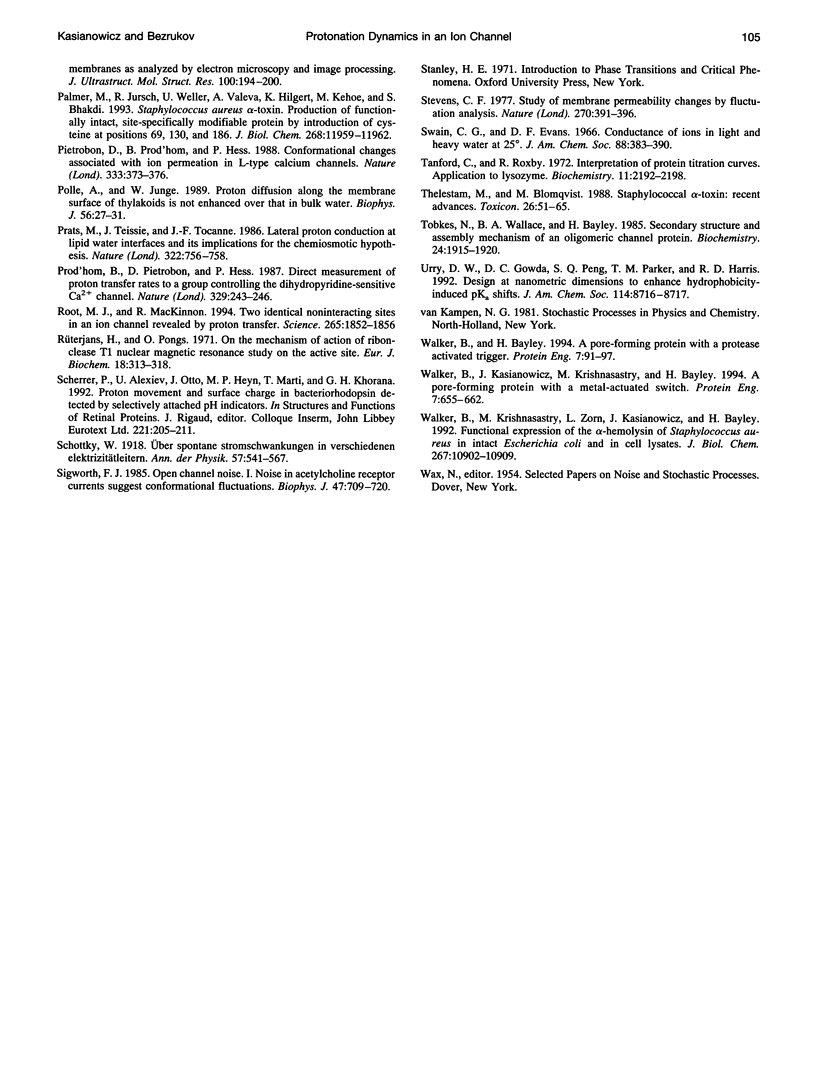
Images in this article
Selected References
These references are in PubMed. This may not be the complete list of references from this article.
- Bayley H. Triggers and switches in a self-assembling pore-forming protein. J Cell Biochem. 1994 Oct;56(2):177–182. doi: 10.1002/jcb.240560210. [DOI] [PubMed] [Google Scholar]
- Bean R. C., Shepherd W. C., Chan H., Eichner J. Discrete conductance fluctuations in lipid bilayer protein membranes. J Gen Physiol. 1969 Jun;53(6):741–757. doi: 10.1085/jgp.53.6.741. [DOI] [PMC free article] [PubMed] [Google Scholar]
- Bezrukov SM, Kasianowicz JJ. Current noise reveals protonation kinetics and number of ionizable sites in an open protein ion channel. Phys Rev Lett. 1993 Apr 12;70(15):2352–2355. doi: 10.1103/PhysRevLett.70.2352. [DOI] [PubMed] [Google Scholar]
- Bhakdi S., Füssle R., Tranum-Jensen J. Staphylococcal alpha-toxin: oligomerization of hydrophilic monomers to form amphiphilic hexamers induced through contact with deoxycholate detergent micelles. Proc Natl Acad Sci U S A. 1981 Sep;78(9):5475–5479. doi: 10.1073/pnas.78.9.5475. [DOI] [PMC free article] [PubMed] [Google Scholar]
- Bhakdi S., Muhly M., Füssle R. Correlation between toxin binding and hemolytic activity in membrane damage by staphylococcal alpha-toxin. Infect Immun. 1984 Nov;46(2):318–323. doi: 10.1128/iai.46.2.318-323.1984. [DOI] [PMC free article] [PubMed] [Google Scholar]
- Bhakdi S., Tranum-Jensen J. Alpha-toxin of Staphylococcus aureus. Microbiol Rev. 1991 Dec;55(4):733–751. doi: 10.1128/mr.55.4.733-751.1991. [DOI] [PMC free article] [PubMed] [Google Scholar]
- Blachly-Dyson E., Peng S., Colombini M., Forte M. Selectivity changes in site-directed mutants of the VDAC ion channel: structural implications. Science. 1990 Mar 9;247(4947):1233–1236. doi: 10.1126/science.1690454. [DOI] [PubMed] [Google Scholar]
- Cescatti L., Pederzolli C., Menestrina G. Modification of lysine residues of Staphylococcus aureus alpha-toxin: effects on its channel-forming properties. J Membr Biol. 1991 Jan;119(1):53–64. doi: 10.1007/BF01868540. [DOI] [PubMed] [Google Scholar]
- Chattopadhyay A., London E. Spectroscopic and ionization properties of N-(7-nitrobenz-2-oxa-1,3-diazol-4-yl)-labeled lipids in model membranes. Biochim Biophys Acta. 1988 Feb 8;938(1):24–34. doi: 10.1016/0005-2736(88)90118-6. [DOI] [PubMed] [Google Scholar]
- Cohen J. S., Shrager R. I., McNeel M., Schnechter A. N. On-line computer-assisted analysis of 220 MHz NMR data of protin imidazole resonances. Biochem Biophys Res Commun. 1970 Jul 13;40(1):144–151. doi: 10.1016/0006-291x(70)91058-2. [DOI] [PubMed] [Google Scholar]
- Deamer D. W., Nichols J. W. Proton flux mechanisms in model and biological membranes. J Membr Biol. 1989 Feb;107(2):91–103. doi: 10.1007/BF01871715. [DOI] [PubMed] [Google Scholar]
- Füssle R., Bhakdi S., Sziegoleit A., Tranum-Jensen J., Kranz T., Wellensiek H. J. On the mechanism of membrane damage by Staphylococcus aureus alpha-toxin. J Cell Biol. 1981 Oct;91(1):83–94. doi: 10.1083/jcb.91.1.83. [DOI] [PMC free article] [PubMed] [Google Scholar]
- Gilson M. K., Honig B. H. Calculation of electrostatic potentials in an enzyme active site. Nature. 1987 Nov 5;330(6143):84–86. doi: 10.1038/330084a0. [DOI] [PubMed] [Google Scholar]
- Goto Y., Hagihara Y., Hamada D., Hoshino M., Nishii I. Acid-induced unfolding and refolding transitions of cytochrome c: a three-state mechanism in H2O and D2O. Biochemistry. 1993 Nov 9;32(44):11878–11885. doi: 10.1021/bi00095a017. [DOI] [PubMed] [Google Scholar]
- Gouaux J. E., Braha O., Hobaugh M. R., Song L., Cheley S., Shustak C., Bayley H. Subunit stoichiometry of staphylococcal alpha-hemolysin in crystals and on membranes: a heptameric transmembrane pore. Proc Natl Acad Sci U S A. 1994 Dec 20;91(26):12828–12831. doi: 10.1073/pnas.91.26.12828. [DOI] [PMC free article] [PubMed] [Google Scholar]
- Gray G. S., Kehoe M. Primary sequence of the alpha-toxin gene from Staphylococcus aureus wood 46. Infect Immun. 1984 Nov;46(2):615–618. doi: 10.1128/iai.46.2.615-618.1984. [DOI] [PMC free article] [PubMed] [Google Scholar]
- Grigorjev P. A., Bezrukov S. M. Hofmeister effect in ion transport: reversible binding of halide anions to the roflamycoin channel. Biophys J. 1994 Dec;67(6):2265–2271. doi: 10.1016/S0006-3495(94)80711-8. [DOI] [PMC free article] [PubMed] [Google Scholar]
- Gutman M., Kotlyar A. B., Borovok N., Nachliel E. Reaction of bulk protons with a mitochondrial inner membrane preparation: time-resolved measurements and their analysis. Biochemistry. 1993 Mar 30;32(12):2942–2946. doi: 10.1021/bi00063a003. [DOI] [PubMed] [Google Scholar]
- Gutman M., Nachliel E., Kiryati S. Dynamic studies of proton diffusion in mesoscopic heterogeneous matrix: II. The interbilayer space between phospholipid membranes. Biophys J. 1992 Jul;63(1):281–290. doi: 10.1016/S0006-3495(92)81585-0. [DOI] [PMC free article] [PubMed] [Google Scholar]
- Gutman M., Tsfadia Y., Masad A., Nachliel E. Quantitation of physical-chemical properties of the aqueous phase inside the phoE ionic channel. Biochim Biophys Acta. 1992 Aug 24;1109(2):141–148. doi: 10.1016/0005-2736(92)90077-y. [DOI] [PubMed] [Google Scholar]
- Haines T. H. Anionic lipid headgroups as a proton-conducting pathway along the surface of membranes: a hypothesis. Proc Natl Acad Sci U S A. 1983 Jan;80(1):160–164. doi: 10.1073/pnas.80.1.160. [DOI] [PMC free article] [PubMed] [Google Scholar]
- Heberle J., Dencher N. A. Surface-bound optical probes monitor protein translocation and surface potential changes during the bacteriorhodopsin photocycle. Proc Natl Acad Sci U S A. 1992 Jul 1;89(13):5996–6000. doi: 10.1073/pnas.89.13.5996. [DOI] [PMC free article] [PubMed] [Google Scholar]
- Heberle J., Riesle J., Thiedemann G., Oesterhelt D., Dencher N. A. Proton migration along the membrane surface and retarded surface to bulk transfer. Nature. 1994 Aug 4;370(6488):379–382. doi: 10.1038/370379a0. [DOI] [PubMed] [Google Scholar]
- Heinemann S. H., Sigworth F. J. Open channel noise. V. Fluctuating barriers to ion entry in gramicidin A channels. Biophys J. 1990 Mar;57(3):499–514. doi: 10.1016/S0006-3495(90)82566-2. [DOI] [PMC free article] [PubMed] [Google Scholar]
- Ikigai H., Nakae T. Assembly of the alpha-toxin-hexamer of Staphylococcus aureus in the liposome membrane. J Biol Chem. 1987 Feb 15;262(5):2156–2160. [PubMed] [Google Scholar]
- Jap B. K. Molecular design of PhoE porin and its functional consequences. J Mol Biol. 1989 Jan 20;205(2):407–419. doi: 10.1016/0022-2836(89)90351-3. [DOI] [PubMed] [Google Scholar]
- Kasianowicz J., Benz R., McLaughlin S. How do protons cross the membrane-solution interface? Kinetic studies on bilayer membranes exposed to the protonophore S-13 (5-chloro-3-tert-butyl-2'-chloro-4' nitrosalicylanilide). J Membr Biol. 1987;95(1):73–89. doi: 10.1007/BF01869632. [DOI] [PubMed] [Google Scholar]
- Kell D. B. On the functional proton current pathway of electron transport phosphorylation. An electrodic view. Biochim Biophys Acta. 1979 Jul 3;549(1):55–99. doi: 10.1016/0304-4173(79)90018-1. [DOI] [PubMed] [Google Scholar]
- Krasilnikov O. V., Sabirov R. Z., Ternovsky V. I., Merzliak P. G., Muratkhodjaev J. N. A simple method for the determination of the pore radius of ion channels in planar lipid bilayer membranes. FEMS Microbiol Immunol. 1992 Sep;5(1-3):93–100. doi: 10.1111/j.1574-6968.1992.tb05891.x. [DOI] [PubMed] [Google Scholar]
- MITCHELL P. Coupling of phosphorylation to electron and hydrogen transfer by a chemi-osmotic type of mechanism. Nature. 1961 Jul 8;191:144–148. doi: 10.1038/191144a0. [DOI] [PubMed] [Google Scholar]
- Menestrina G. Ionic channels formed by Staphylococcus aureus alpha-toxin: voltage-dependent inhibition by divalent and trivalent cations. J Membr Biol. 1986;90(2):177–190. doi: 10.1007/BF01869935. [DOI] [PubMed] [Google Scholar]
- Montal M., Mueller P. Formation of bimolecular membranes from lipid monolayers and a study of their electrical properties. Proc Natl Acad Sci U S A. 1972 Dec;69(12):3561–3566. doi: 10.1073/pnas.69.12.3561. [DOI] [PMC free article] [PubMed] [Google Scholar]
- Neher E., Sakmann B. Single-channel currents recorded from membrane of denervated frog muscle fibres. Nature. 1976 Apr 29;260(5554):799–802. doi: 10.1038/260799a0. [DOI] [PubMed] [Google Scholar]
- Neumcke B. Fluctuation of Na and K currents in excitable membranes. Int Rev Neurobiol. 1982;23:35–67. doi: 10.1016/s0074-7742(08)60621-2. [DOI] [PubMed] [Google Scholar]
- Olofsson A., Kavéus U., Thelestam M., Hebert H. The projection structure of alpha-toxin from Staphylococcus aureus in human platelet membranes as analyzed by electron microscopy and image processing. J Ultrastruct Mol Struct Res. 1988 Aug;100(2):194–200. doi: 10.1016/0889-1605(88)90026-2. [DOI] [PubMed] [Google Scholar]
- Palmer M., Jursch R., Weller U., Valeva A., Hilgert K., Kehoe M., Bhakdi S. Staphylococcus aureus alpha-toxin. Production of functionally intact, site-specifically modifiable protein by introduction of cysteine at positions 69, 130, and 186. J Biol Chem. 1993 Jun 5;268(16):11959–11962. [PubMed] [Google Scholar]
- Pietrobon D., Prod'hom B., Hess P. Conformational changes associated with ion permeation in L-type calcium channels. Nature. 1988 May 26;333(6171):373–376. doi: 10.1038/333373a0. [DOI] [PubMed] [Google Scholar]
- Polle A., Junge W. Proton diffusion along the membrane surface of thylakoids is not enhanced over that in bulk water. Biophys J. 1989 Jul;56(1):27–31. doi: 10.1016/S0006-3495(89)82649-9. [DOI] [PMC free article] [PubMed] [Google Scholar]
- Prod'hom B., Pietrobon D., Hess P. Direct measurement of proton transfer rates to a group controlling the dihydropyridine-sensitive Ca2+ channel. Nature. 1987 Sep 17;329(6136):243–246. doi: 10.1038/329243a0. [DOI] [PubMed] [Google Scholar]
- Root M. J., MacKinnon R. Two identical noninteracting sites in an ion channel revealed by proton transfer. Science. 1994 Sep 23;265(5180):1852–1856. doi: 10.1126/science.7522344. [DOI] [PubMed] [Google Scholar]
- Rüterjans H., Pongs O. On the mechanism of action of ribonuclease T1. Nuclear magnetic resonance study on the active site. Eur J Biochem. 1971 Feb 1;18(3):313–318. doi: 10.1111/j.1432-1033.1971.tb01246.x. [DOI] [PubMed] [Google Scholar]
- Sigworth F. J. Open channel noise. I. Noise in acetylcholine receptor currents suggests conformational fluctuations. Biophys J. 1985 May;47(5):709–720. doi: 10.1016/S0006-3495(85)83968-0. [DOI] [PMC free article] [PubMed] [Google Scholar]
- Stevens C. F. Study of membrane permeability changes by fluctuation analysis. Nature. 1977 Dec 1;270(5636):391–396. doi: 10.1038/270391a0. [DOI] [PubMed] [Google Scholar]
- Tanford C., Roxby R. Interpretation of protein titration curves. Application to lysozyme. Biochemistry. 1972 May 23;11(11):2192–2198. doi: 10.1021/bi00761a029. [DOI] [PubMed] [Google Scholar]
- Thelestam M., Blomqvist L. Staphylococcal alpha toxin--recent advances. Toxicon. 1988;26(1):55–65. doi: 10.1016/0041-0101(88)90137-7. [DOI] [PubMed] [Google Scholar]
- Tobkes N., Wallace B. A., Bayley H. Secondary structure and assembly mechanism of an oligomeric channel protein. Biochemistry. 1985 Apr 9;24(8):1915–1920. doi: 10.1021/bi00329a017. [DOI] [PubMed] [Google Scholar]
- Walker B., Bayley H. A pore-forming protein with a protease-activated trigger. Protein Eng. 1994 Jan;7(1):91–97. doi: 10.1093/protein/7.1.91. [DOI] [PubMed] [Google Scholar]
- Walker B., Kasianowicz J., Krishnasastry M., Bayley H. A pore-forming protein with a metal-actuated switch. Protein Eng. 1994 May;7(5):655–662. doi: 10.1093/protein/7.5.655. [DOI] [PubMed] [Google Scholar]
- Walker B., Krishnasastry M., Zorn L., Kasianowicz J., Bayley H. Functional expression of the alpha-hemolysin of Staphylococcus aureus in intact Escherichia coli and in cell lysates. Deletion of five C-terminal amino acids selectively impairs hemolytic activity. J Biol Chem. 1992 May 25;267(15):10902–10909. [PubMed] [Google Scholar]