Abstract
At high temperature, nicked free monomers of diphtheria toxin undergo a transition to a thermally denatured state, with a midpoint of 45-50 degrees C. In this report, the high-temperature (60 degrees C) conformation has been compared to the native (neutral pH) and low-pH (pH less than 5) conformations. The low-pH and high-temperature conformations are similar although not identical. As in the conformation at low pH, and unlike the toxin in its native conformation, the protein in its conformation at high temperature is hydrophobic, has low fluorescence intensity, and has increased exposure of tryptophan to aqueous solution. As at low pH, at high temperature the circular dichroism spectrum shows at most only partial unfolding of secondary structure. In contrast, the conformation of the toxin in guanidinium chloride is much closer to a random coil. The effects of high temperature and low pH interact in the sense that sensitivity of the native conformation to one is increased by the other. That is, the transition temperature between native and thermally denatured states is decreased as pH is decreased, and the transition pH between neutral-pH and low-pH states is increased as temperature is increased. This implies that there is some region on the protein where high temperature and low pH can disrupt conformation in a similar manner. Taken together, these results indicate that the low-pH and high-temperature conformations can both be defined as partially denatured states, even though unfolding may not be extensive at low pH. Similar behavior may occur in other proteins that undergo functionally important conformational disruption at low pH.
Full text
PDF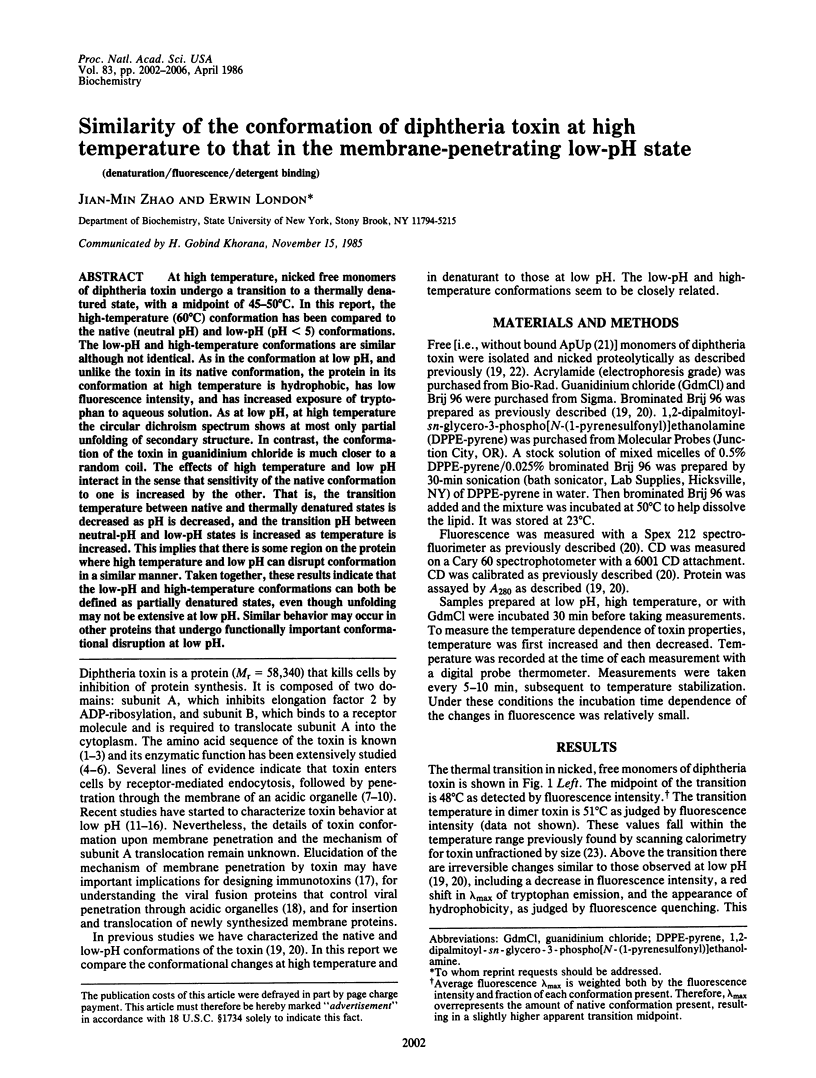
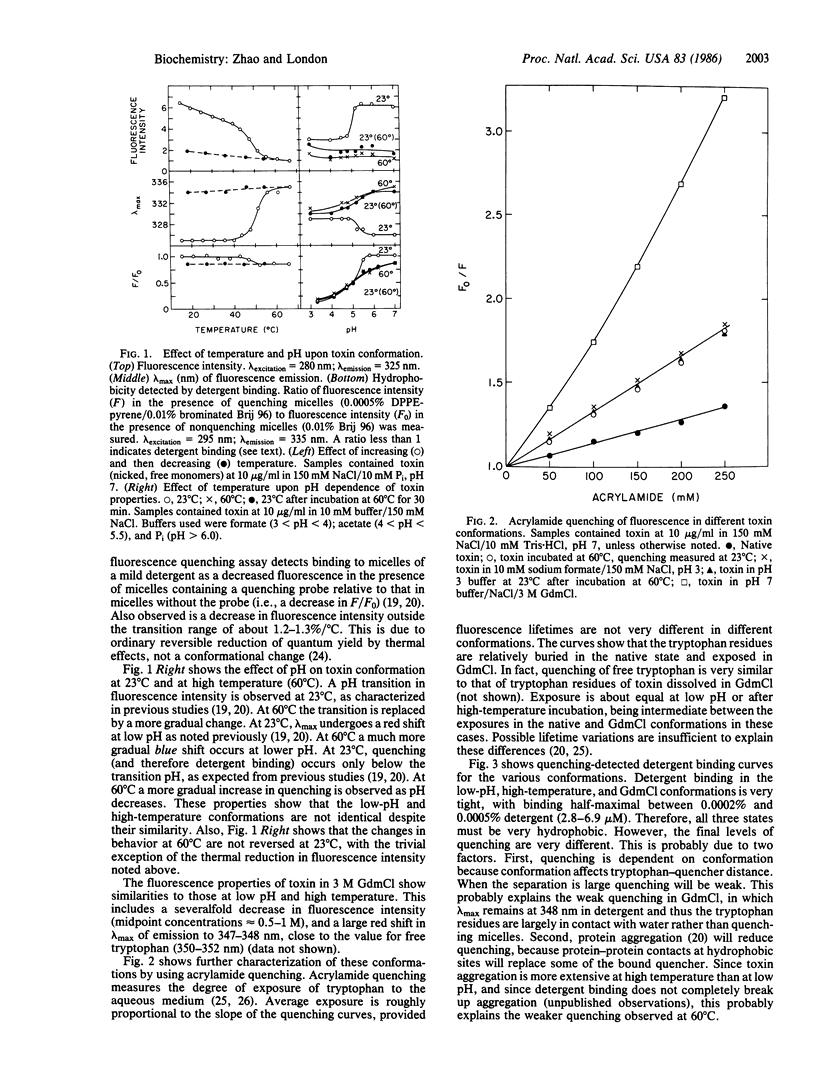
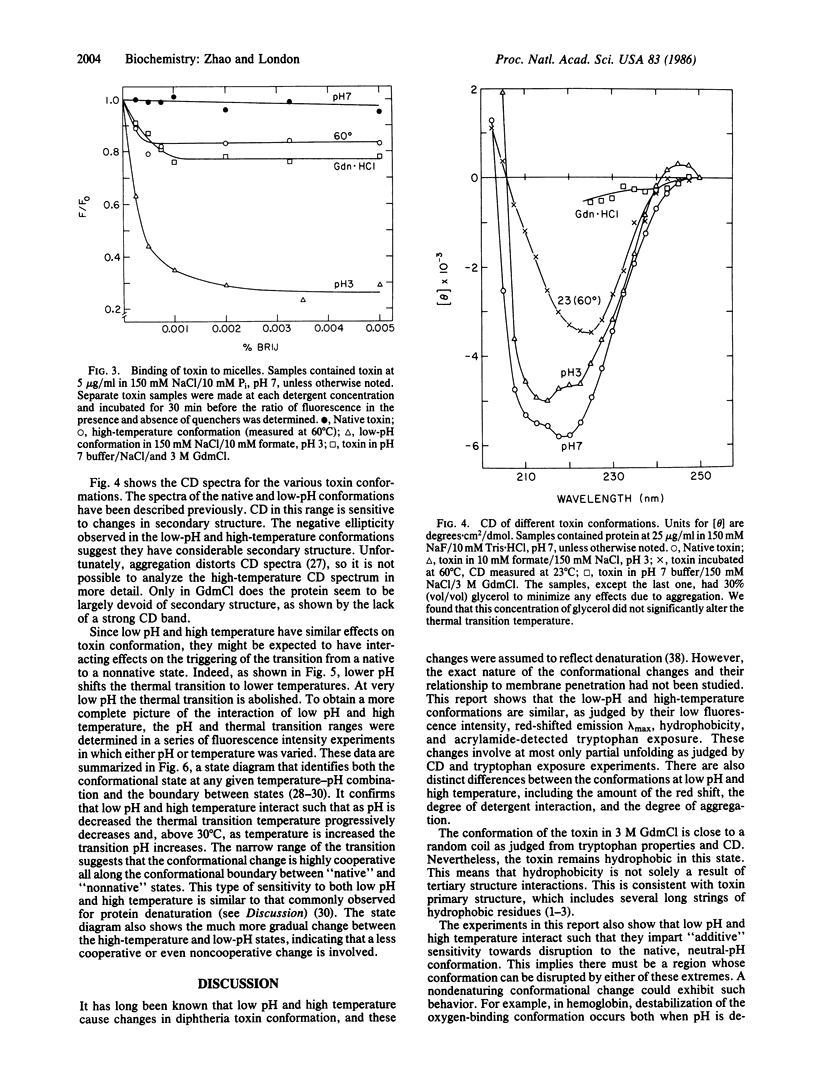
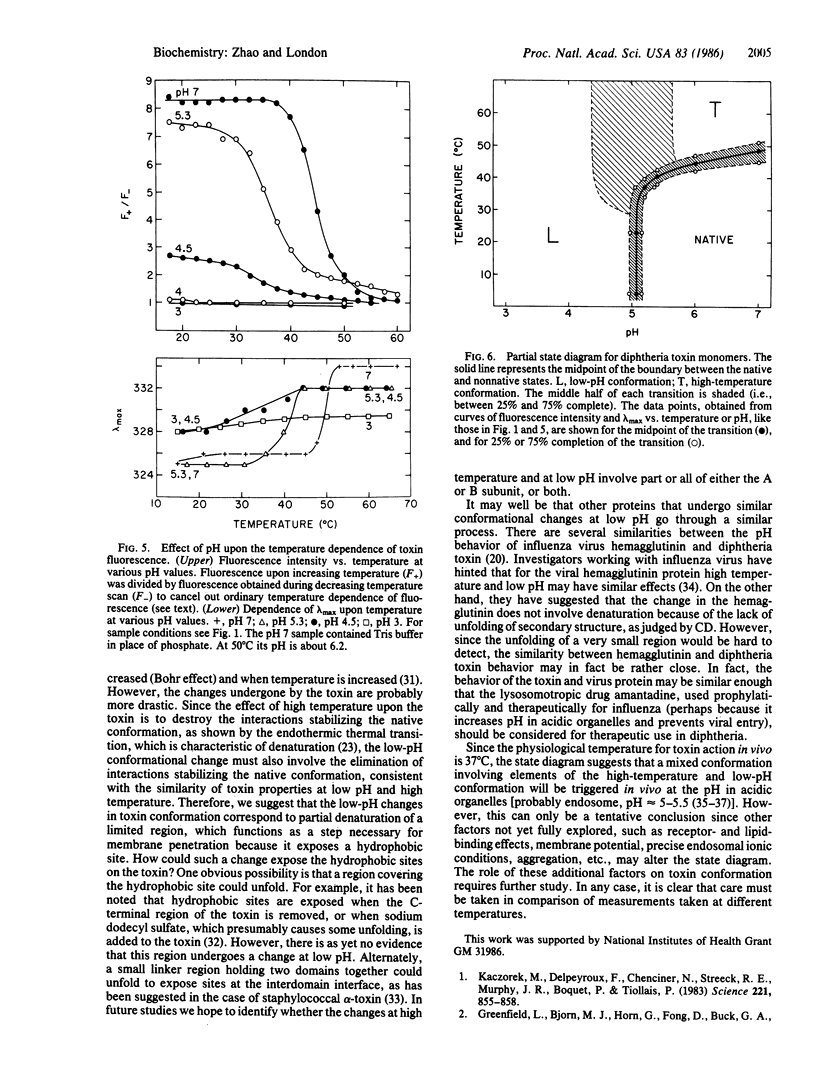
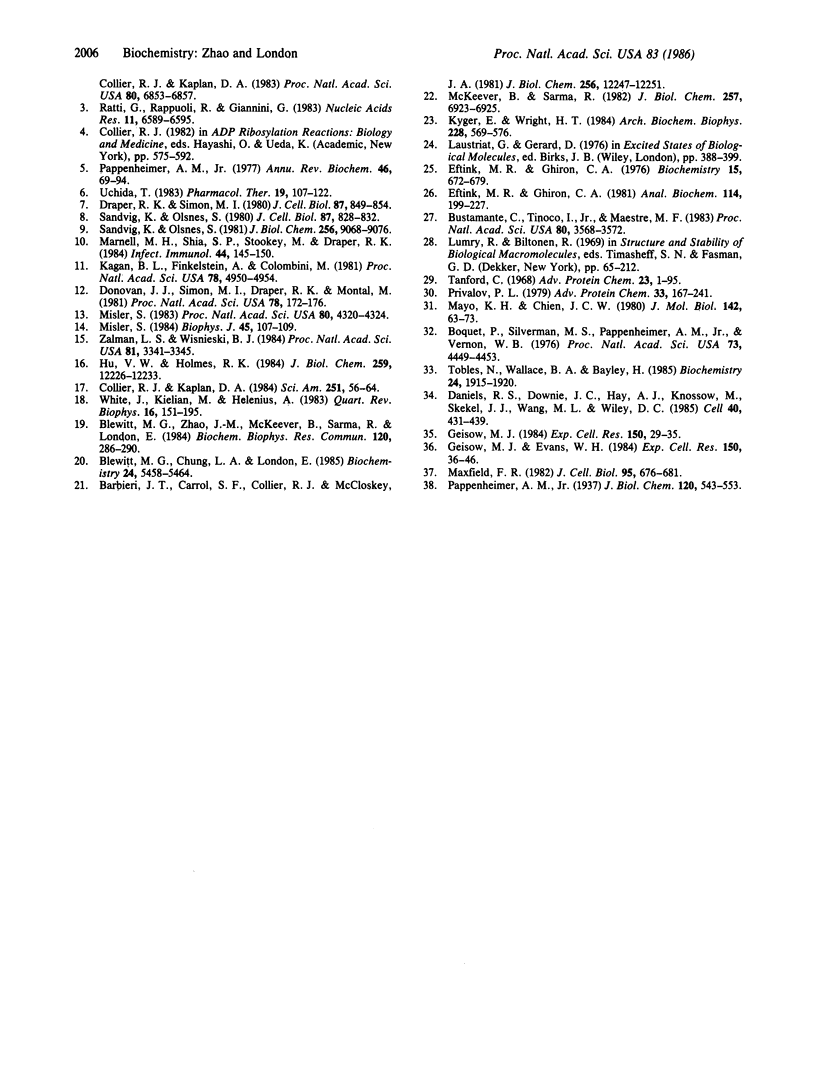
Selected References
These references are in PubMed. This may not be the complete list of references from this article.
- Barbieri J. T., Carroll S. F., Collier R. J., McCloskey J. A. An endogenous dinucleotide bound to diphtheria toxin. Adenylyl-(3',5')-uridine 3'-monophosphate. J Biol Chem. 1981 Dec 10;256(23):12247–12251. [PubMed] [Google Scholar]
- Blewitt M. G., Chao J. M., McKeever B., Sarma R., London E. Fluorescence characterization of the low pH-induced change in diphtheria toxin conformation: effect of salt. Biochem Biophys Res Commun. 1984 Apr 16;120(1):286–290. doi: 10.1016/0006-291x(84)91446-3. [DOI] [PubMed] [Google Scholar]
- Blewitt M. G., Chung L. A., London E. Effect of pH on the conformation of diphtheria toxin and its implications for membrane penetration. Biochemistry. 1985 Sep 24;24(20):5458–5464. doi: 10.1021/bi00341a027. [DOI] [PubMed] [Google Scholar]
- Boquet P., Silverman M. S., Pappenheimer A. M., Jr, Vernon W. B. Binding of triton X-100 to diphtheria toxin, crossreacting material 45, and their fragments. Proc Natl Acad Sci U S A. 1976 Dec;73(12):4449–4453. doi: 10.1073/pnas.73.12.4449. [DOI] [PMC free article] [PubMed] [Google Scholar]
- Bustamante C., Tinoco I., Jr, Maestre M. F. Circular differential scattering can be an important part of the circular dichroism of macromolecules. Proc Natl Acad Sci U S A. 1983 Jun;80(12):3568–3572. doi: 10.1073/pnas.80.12.3568. [DOI] [PMC free article] [PubMed] [Google Scholar]
- Collier R. J., Kaplan D. A. Immunotoxins. Sci Am. 1984 Jul;251(1):56–64. doi: 10.1038/scientificamerican0784-56. [DOI] [PubMed] [Google Scholar]
- Daniels R. S., Downie J. C., Hay A. J., Knossow M., Skehel J. J., Wang M. L., Wiley D. C. Fusion mutants of the influenza virus hemagglutinin glycoprotein. Cell. 1985 Feb;40(2):431–439. doi: 10.1016/0092-8674(85)90157-6. [DOI] [PubMed] [Google Scholar]
- Donovan J. J., Simon M. I., Draper R. K., Montal M. Diphtheria toxin forms transmembrane channels in planar lipid bilayers. Proc Natl Acad Sci U S A. 1981 Jan;78(1):172–176. doi: 10.1073/pnas.78.1.172. [DOI] [PMC free article] [PubMed] [Google Scholar]
- Draper R. K., Simon M. I. The entry of diphtheria toxin into the mammalian cell cytoplasm: evidence for lysosomal involvement. J Cell Biol. 1980 Dec;87(3 Pt 1):849–854. doi: 10.1083/jcb.87.3.849. [DOI] [PMC free article] [PubMed] [Google Scholar]
- Eftink M. R., Ghiron C. A. Exposure of tryptophanyl residues in proteins. Quantitative determination by fluorescence quenching studies. Biochemistry. 1976 Feb 10;15(3):672–680. doi: 10.1021/bi00648a035. [DOI] [PubMed] [Google Scholar]
- Eftink M. R., Ghiron C. A. Fluorescence quenching studies with proteins. Anal Biochem. 1981 Jul 1;114(2):199–227. doi: 10.1016/0003-2697(81)90474-7. [DOI] [PubMed] [Google Scholar]
- Geisow M. J., Evans W. H. pH in the endosome. Measurements during pinocytosis and receptor-mediated endocytosis. Exp Cell Res. 1984 Jan;150(1):36–46. doi: 10.1016/0014-4827(84)90699-2. [DOI] [PubMed] [Google Scholar]
- Geisow M. J. Fluorescein conjugates as indicators of subcellular pH. A critical evaluation. Exp Cell Res. 1984 Jan;150(1):29–35. doi: 10.1016/0014-4827(84)90698-0. [DOI] [PubMed] [Google Scholar]
- Greenfield L., Bjorn M. J., Horn G., Fong D., Buck G. A., Collier R. J., Kaplan D. A. Nucleotide sequence of the structural gene for diphtheria toxin carried by corynebacteriophage beta. Proc Natl Acad Sci U S A. 1983 Nov;80(22):6853–6857. doi: 10.1073/pnas.80.22.6853. [DOI] [PMC free article] [PubMed] [Google Scholar]
- Hu V. W., Holmes R. K. Evidence for direct insertion of fragments A and B of diphtheria toxin into model membranes. J Biol Chem. 1984 Oct 10;259(19):12226–12233. [PubMed] [Google Scholar]
- Kaczorek M., Delpeyroux F., Chenciner N., Streeck R. E., Murphy J. R., Boquet P., Tiollais P. Nucleotide sequence and expression of the diphtheria tox228 gene in Escherichia coli. Science. 1983 Aug 26;221(4613):855–858. doi: 10.1126/science.6348945. [DOI] [PubMed] [Google Scholar]
- Kagan B. L., Finkelstein A., Colombini M. Diphtheria toxin fragment forms large pores in phospholipid bilayer membranes. Proc Natl Acad Sci U S A. 1981 Aug;78(8):4950–4954. doi: 10.1073/pnas.78.8.4950. [DOI] [PMC free article] [PubMed] [Google Scholar]
- Kyger E., Wright H. T. Thermal stability of different forms of diphtheria toxin. Arch Biochem Biophys. 1984 Feb 1;228(2):569–576. doi: 10.1016/0003-9861(84)90024-9. [DOI] [PubMed] [Google Scholar]
- Marnell M. H., Shia S. P., Stookey M., Draper R. K. Evidence for penetration of diphtheria toxin to the cytosol through a prelysosomal membrane. Infect Immun. 1984 Apr;44(1):145–150. doi: 10.1128/iai.44.1.145-150.1984. [DOI] [PMC free article] [PubMed] [Google Scholar]
- Maxfield F. R. Weak bases and ionophores rapidly and reversibly raise the pH of endocytic vesicles in cultured mouse fibroblasts. J Cell Biol. 1982 Nov;95(2 Pt 1):676–681. doi: 10.1083/jcb.95.2.676. [DOI] [PMC free article] [PubMed] [Google Scholar]
- Mayo K. H., Chien J. C. Effect of temperature on functional properties of carp hemoglobin. J Mol Biol. 1980 Sep 5;142(1):63–73. doi: 10.1016/0022-2836(80)90206-5. [DOI] [PubMed] [Google Scholar]
- McKeever B., Sarma R. Preliminary crystallographic investigation of the protein toxin from corynebacterium diphtheriae. J Biol Chem. 1982 Jun 25;257(12):6923–6925. [PubMed] [Google Scholar]
- Misler S. Gating of ion channels made by a diphtheria toxin fragment in phospholipid bilayer membranes. Proc Natl Acad Sci U S A. 1983 Jul;80(14):4320–4324. doi: 10.1073/pnas.80.14.4320. [DOI] [PMC free article] [PubMed] [Google Scholar]
- Pappenheimer A. M., Jr Diphtheria toxin. Annu Rev Biochem. 1977;46:69–94. doi: 10.1146/annurev.bi.46.070177.000441. [DOI] [PubMed] [Google Scholar]
- Privalov P. L. Stability of proteins: small globular proteins. Adv Protein Chem. 1979;33:167–241. doi: 10.1016/s0065-3233(08)60460-x. [DOI] [PubMed] [Google Scholar]
- RUBINSTEIN D., RABINOWITCH E. ACTION SPECTRUM FOR THE APPEARANCE OF THE 520 MILLIMICRON DIFFERENCE BAND IN ILLUMINATED CHLORELLA CELLS. Biophys J. 1964 Mar;4:107–113. doi: 10.1016/s0006-3495(64)86772-2. [DOI] [PMC free article] [PubMed] [Google Scholar]
- Ratti G., Rappuoli R., Giannini G. The complete nucleotide sequence of the gene coding for diphtheria toxin in the corynephage omega (tox+) genome. Nucleic Acids Res. 1983 Oct 11;11(19):6589–6595. doi: 10.1093/nar/11.19.6589. [DOI] [PMC free article] [PubMed] [Google Scholar]
- Sandvig K., Olsnes S. Diphtheria toxin entry into cells is facilitated by low pH. J Cell Biol. 1980 Dec;87(3 Pt 1):828–832. doi: 10.1083/jcb.87.3.828. [DOI] [PMC free article] [PubMed] [Google Scholar]
- Sandvig K., Olsnes S. Rapid entry of nicked diphtheria toxin into cells at low pH. Characterization of the entry process and effects of low pH on the toxin molecule. J Biol Chem. 1981 Sep 10;256(17):9068–9076. [PubMed] [Google Scholar]
- Tobkes N., Wallace B. A., Bayley H. Secondary structure and assembly mechanism of an oligomeric channel protein. Biochemistry. 1985 Apr 9;24(8):1915–1920. doi: 10.1021/bi00329a017. [DOI] [PubMed] [Google Scholar]
- Uchida T. Diphtheria toxin. Pharmacol Ther. 1982;19(1):107–122. doi: 10.1016/0163-7258(82)90043-2. [DOI] [PubMed] [Google Scholar]
- White J., Kielian M., Helenius A. Membrane fusion proteins of enveloped animal viruses. Q Rev Biophys. 1983 May;16(2):151–195. doi: 10.1017/s0033583500005072. [DOI] [PubMed] [Google Scholar]
- Zalman L. S., Wisnieski B. J. Mechanism of insertion of diphtheria toxin: peptide entry and pore size determinations. Proc Natl Acad Sci U S A. 1984 Jun;81(11):3341–3345. doi: 10.1073/pnas.81.11.3341. [DOI] [PMC free article] [PubMed] [Google Scholar]