Abstract
Many models of the cytoskeletal motility of Amoeba proteus can be formulated in terms of the theory of reactive interpenetrating flow (Dembo and Harlow, 1986). We have devised numerical methodology for testing such models against the phenomenon of steady axisymmetric fountain flow. The simplest workable scheme revealed by such tests (the minimal model) is the main preoccupation of this study. All parameters of the minimal model are determined from available data. Using these parameters the model quantitatively accounts for the self assembly of the cytoskeleton of A. proteus: for the formation and detailed morphology of the endoplasmic channel, the ectoplasmic tube, the uropod, the plasma gel sheet, and the hyaline cap. The model accounts for the kinematics of the cytoskeleton: the detailed velocity field of the forward flow of the endoplasm, the contraction of the ectoplasmic tube, and the inversion of the flow in the fountain zone. The model also gives a satisfactory account of measurements of pressure gradients, measurements of heat dissipation, and measurements of the output of useful work by amoeba. Finally, the model suggests a very promising (but still hypothetical) continuum formulation of the free boundary problem of amoeboid motion. by balancing normal forces on the plasma membrane as closely as possible, the minimal model is able to predict the turgor pressure and surface tension of A. proteus. Several dynamical factors are crucial to the success of the minimal model and are likely to be general features of cytoskeletal mechanics and control in amoeboid cells. These are: a constitutive law for the viscosity of the contractile network that includes an automatic process of gelation as the network density gets large; a very vigorous cycle of network polymerization and depolymerization (in the case of A. proteus, the time constant for this reaction is approximately 12 s); control of network contractility by a diffusible factor (probably calcium ion); and control of the adhesive interaction between the cytoskeleton and the inner surface of the plasma membrane.
Full text
PDF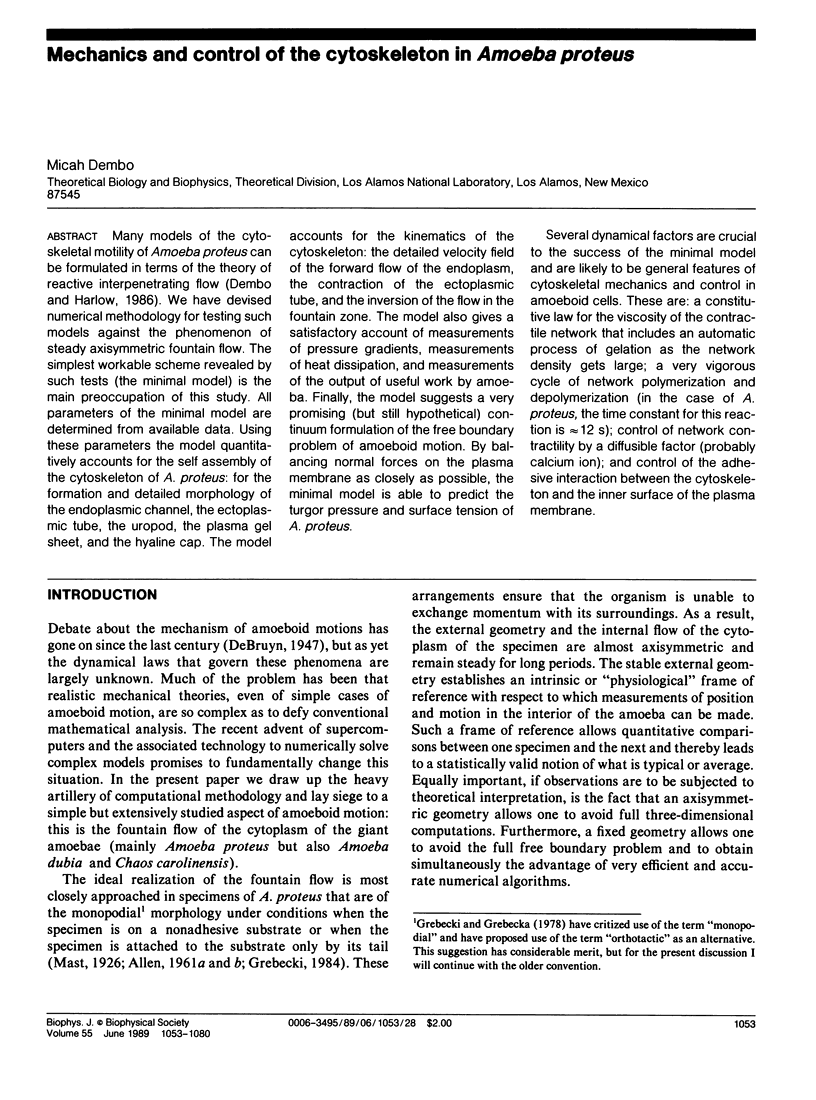
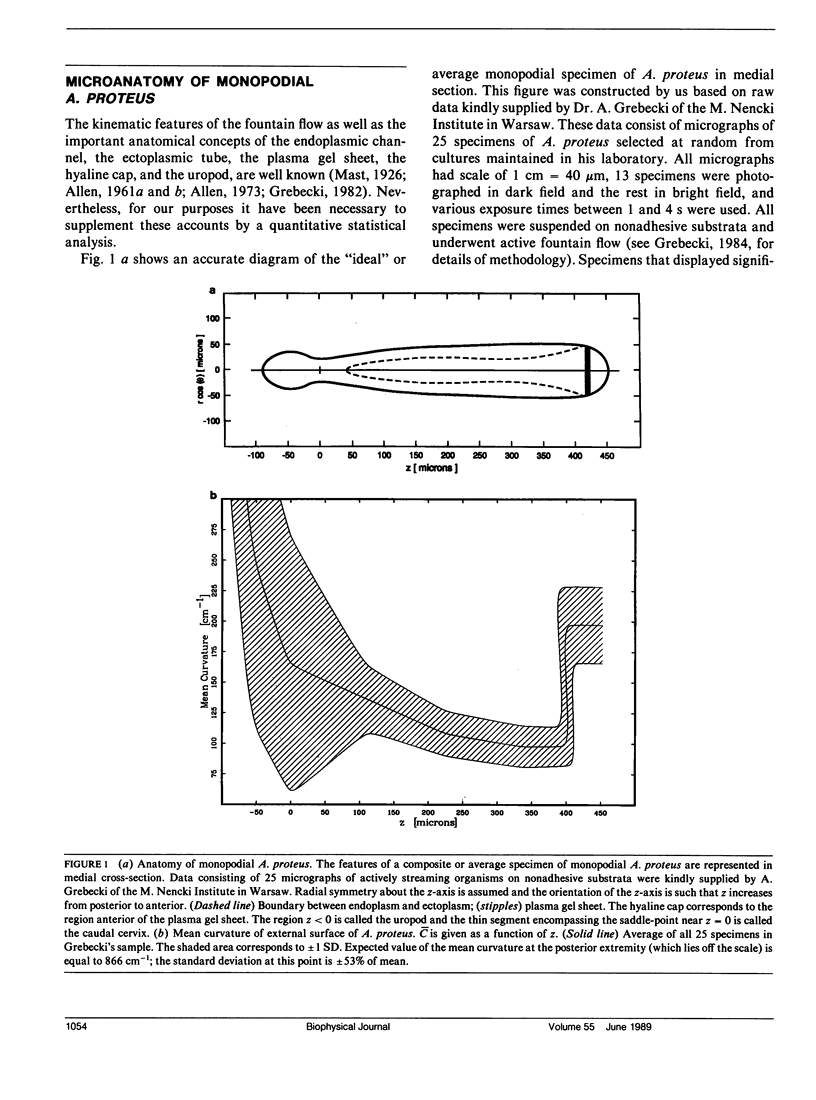
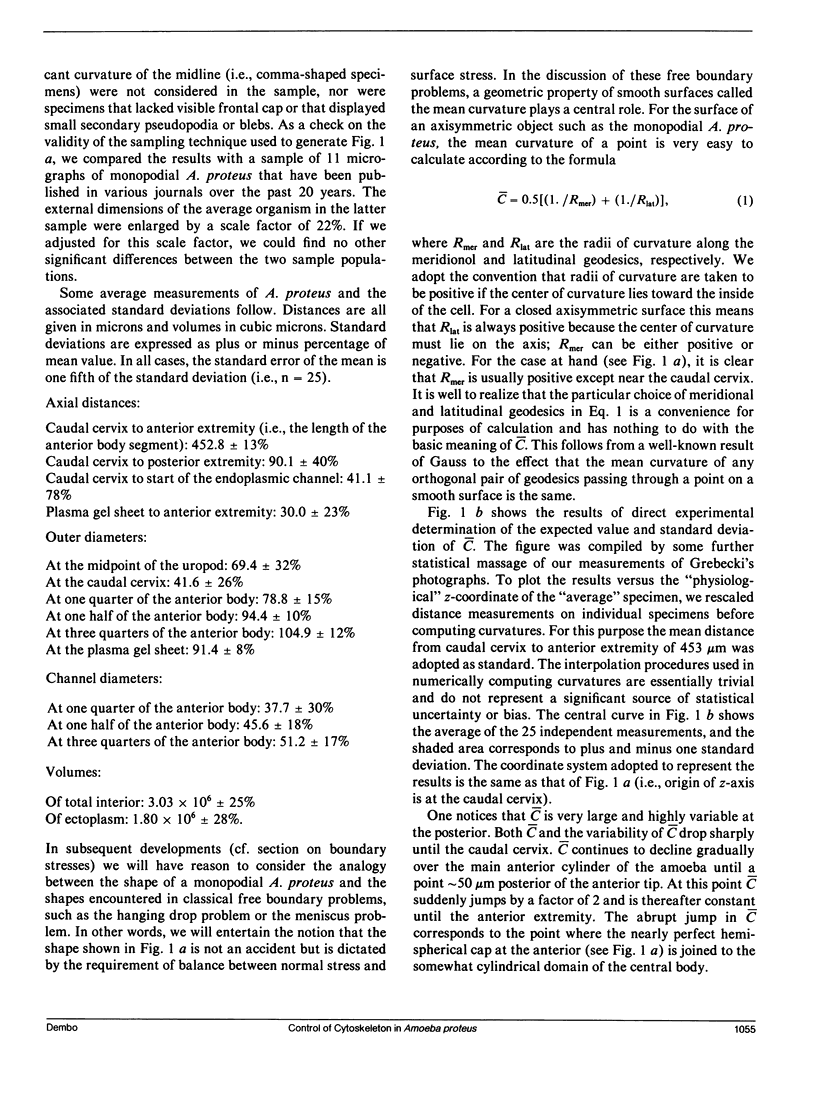
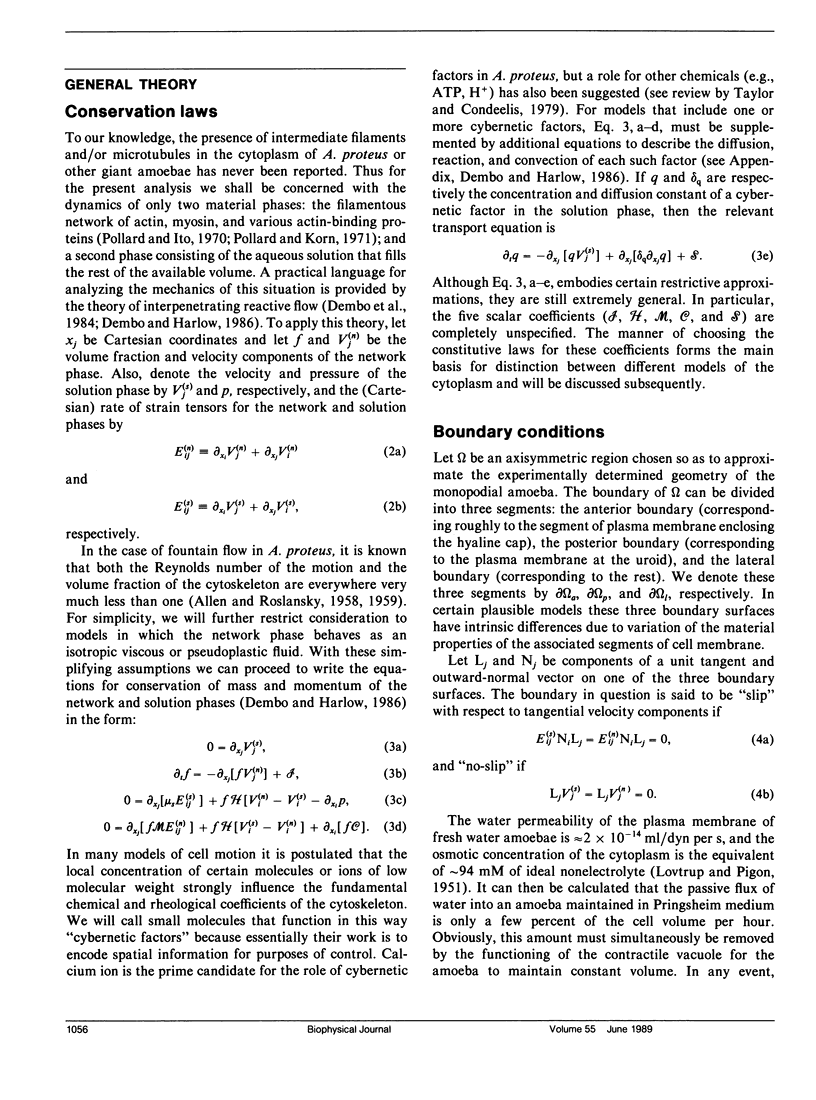
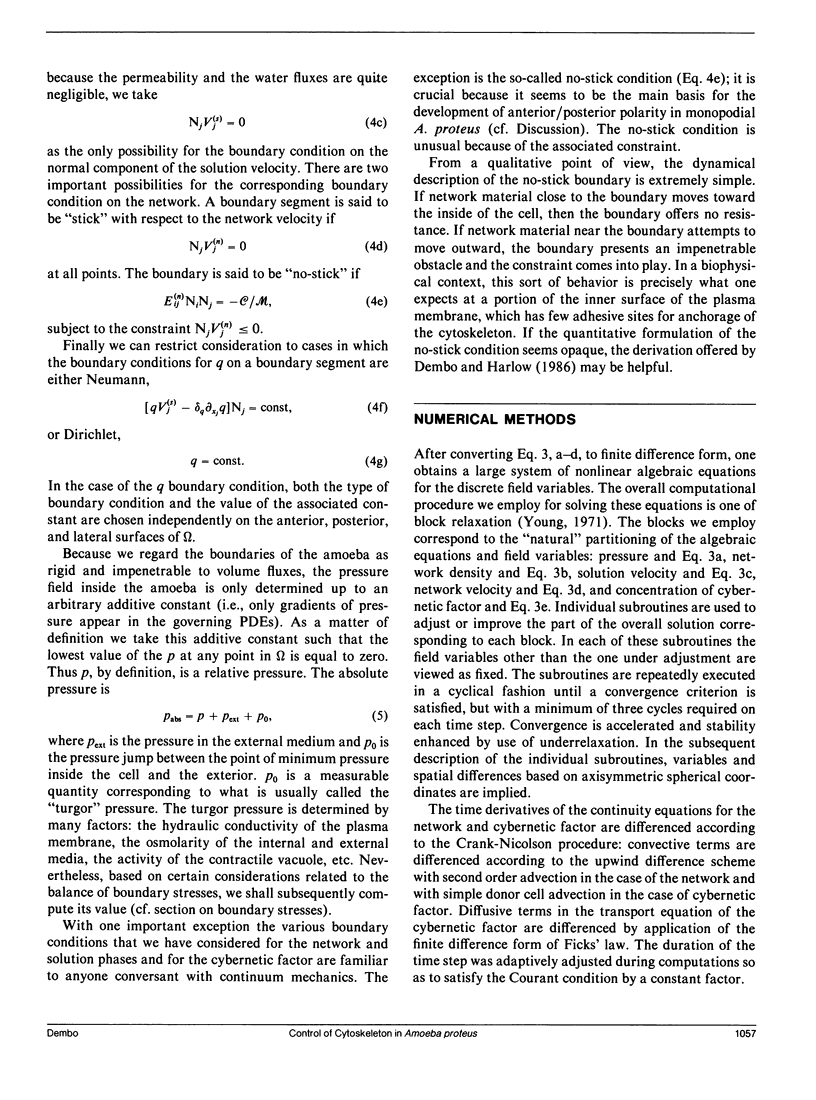
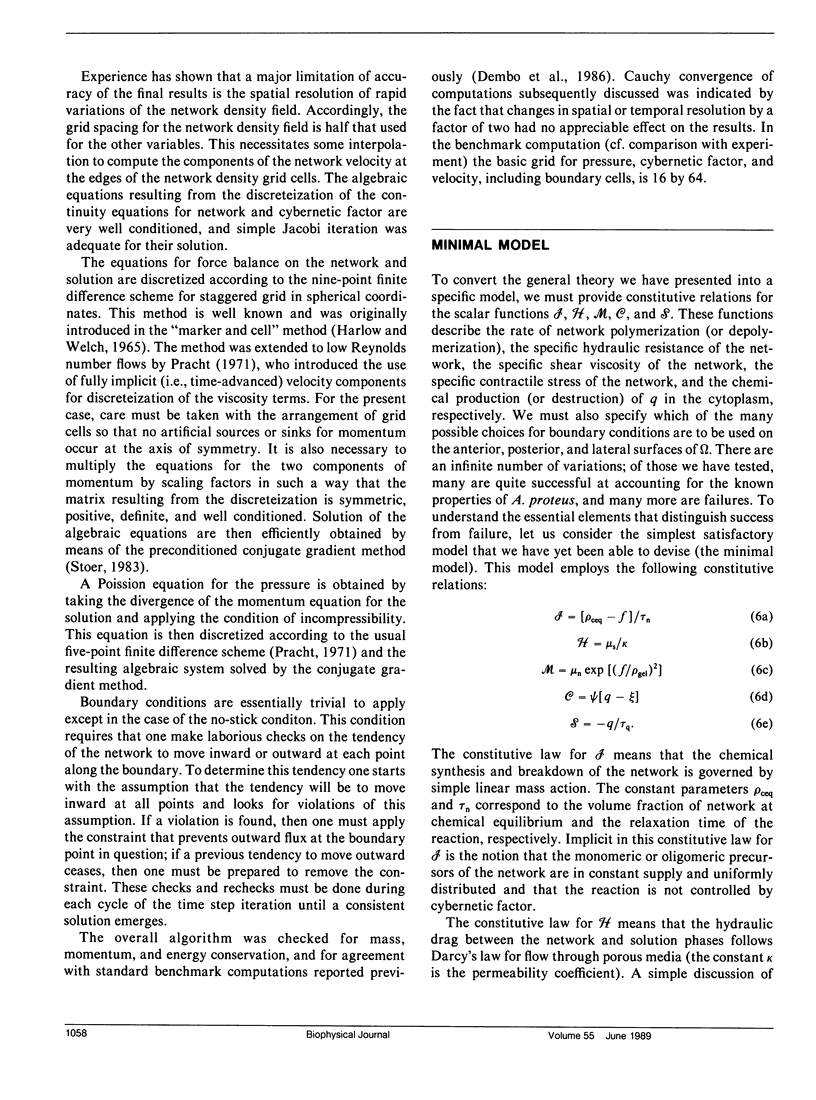
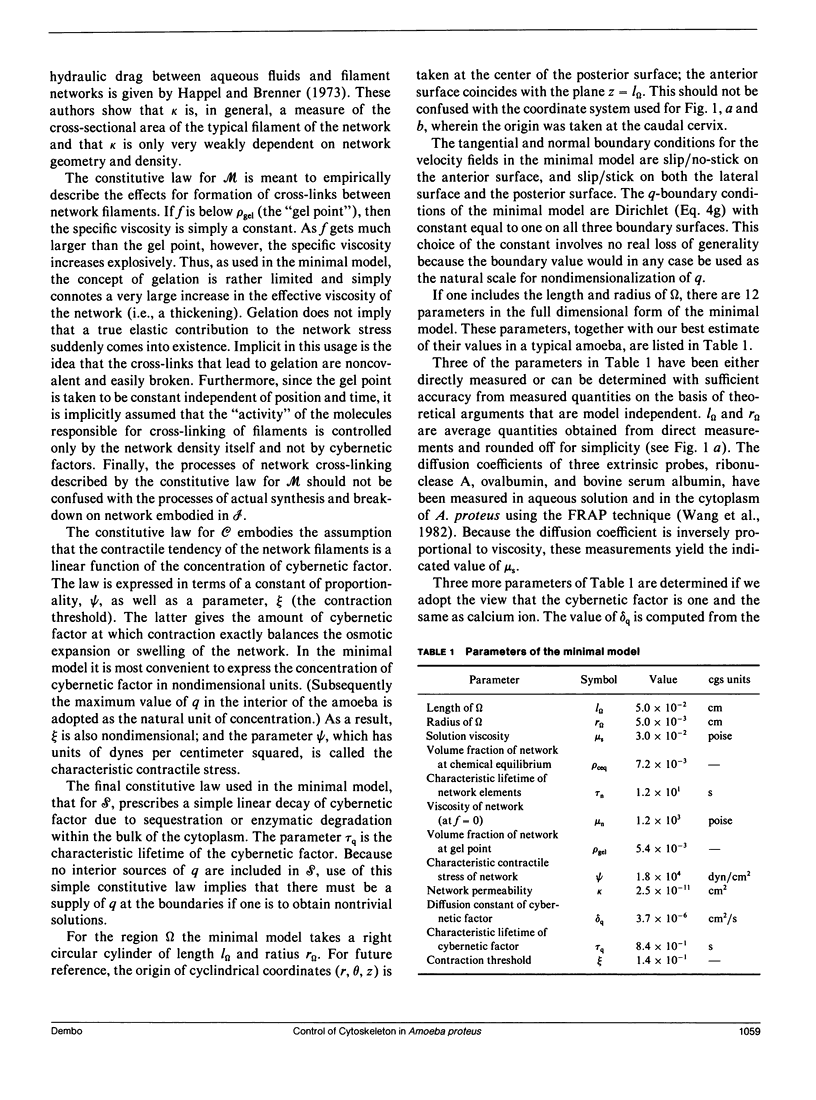
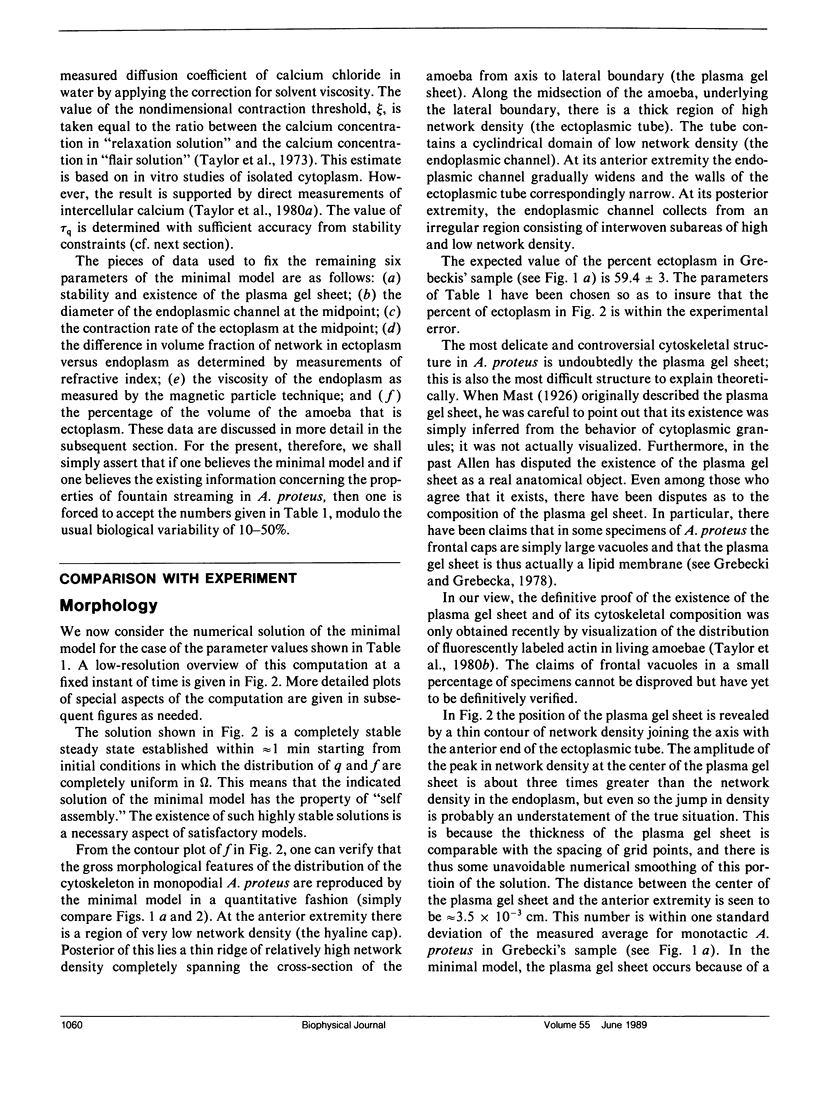
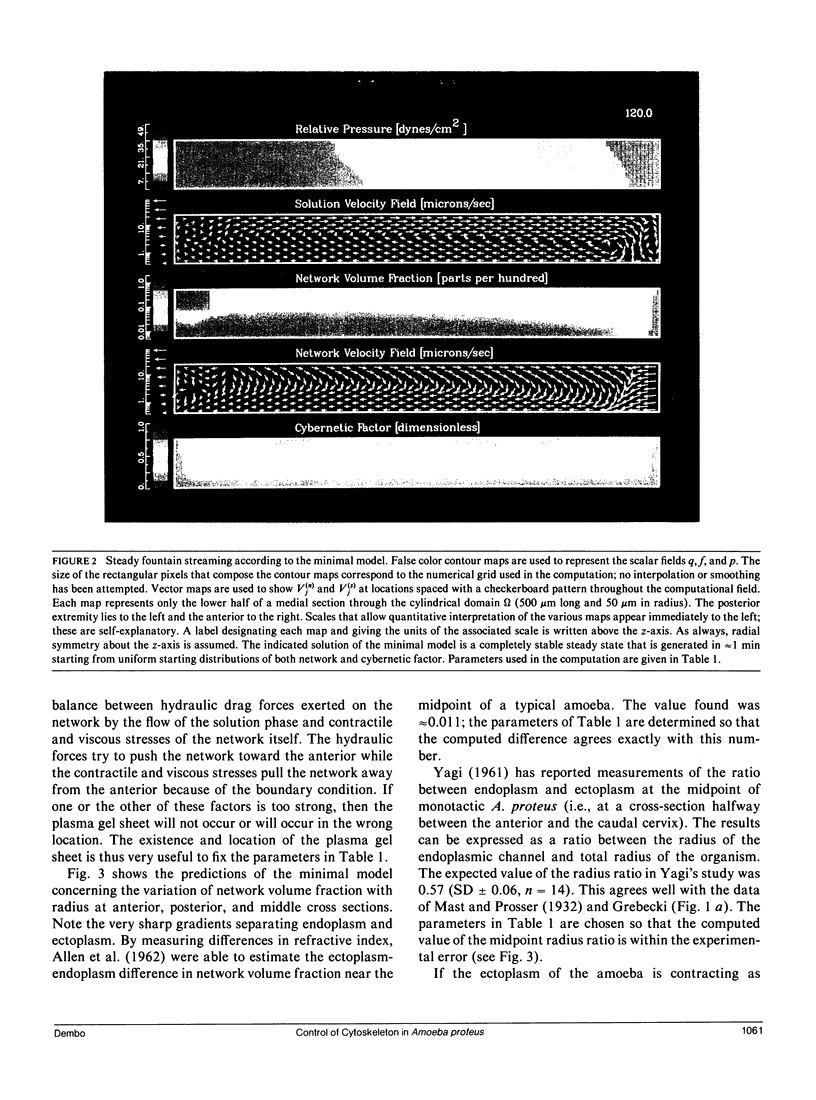
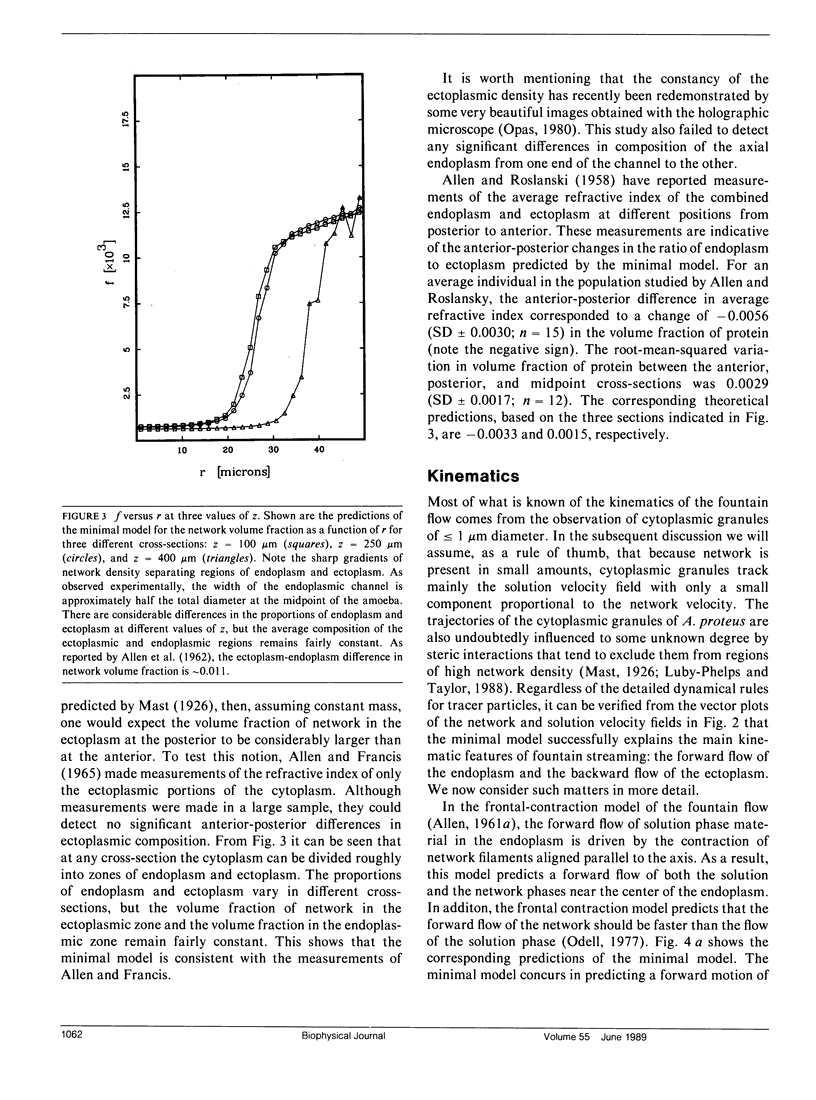
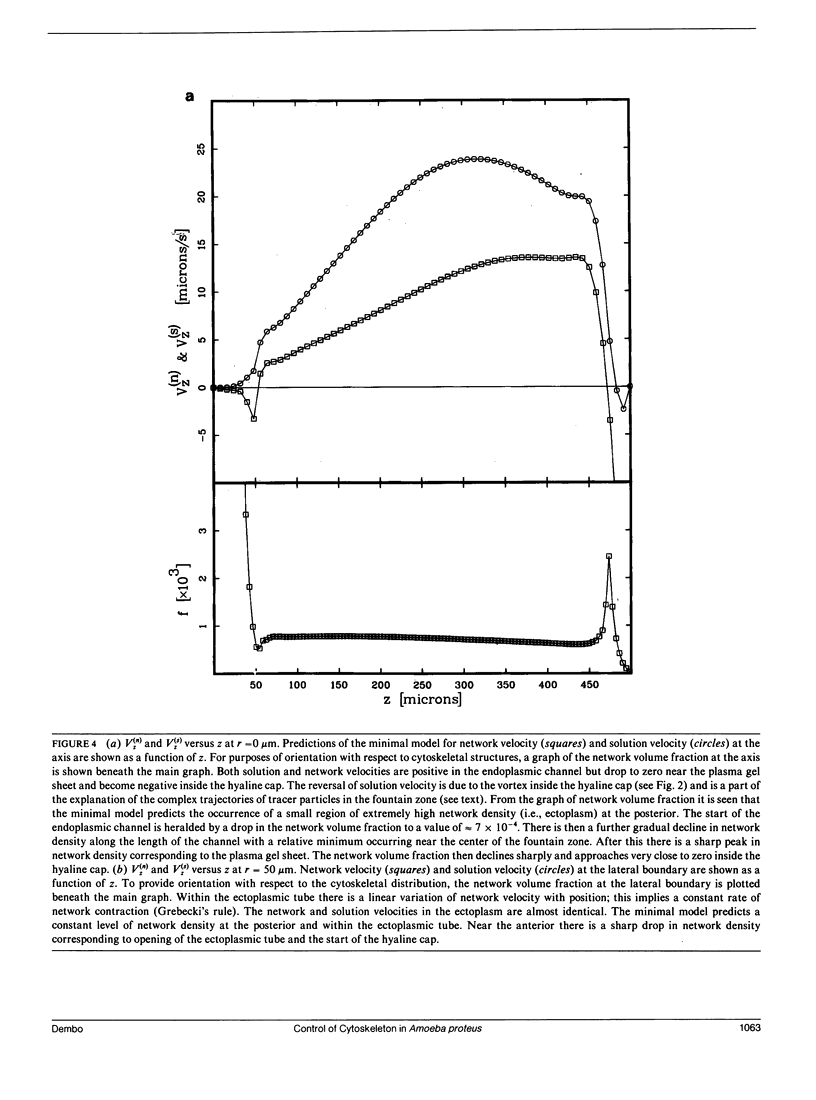
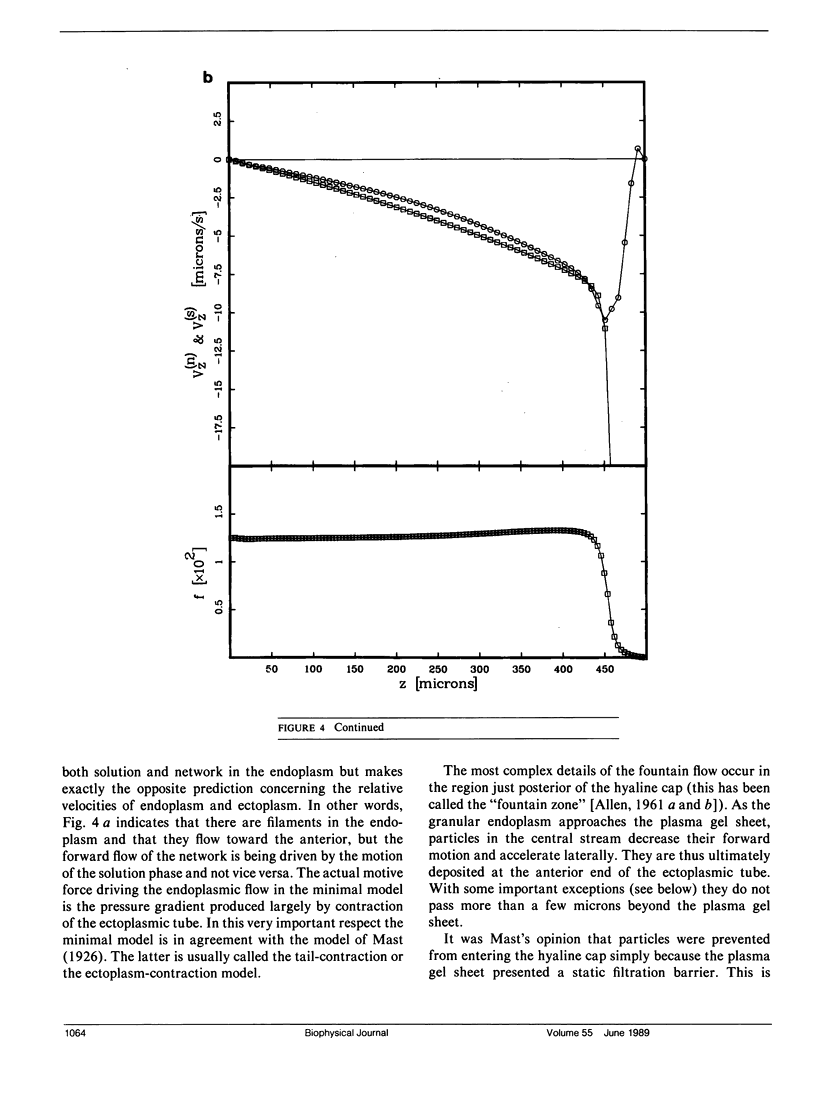
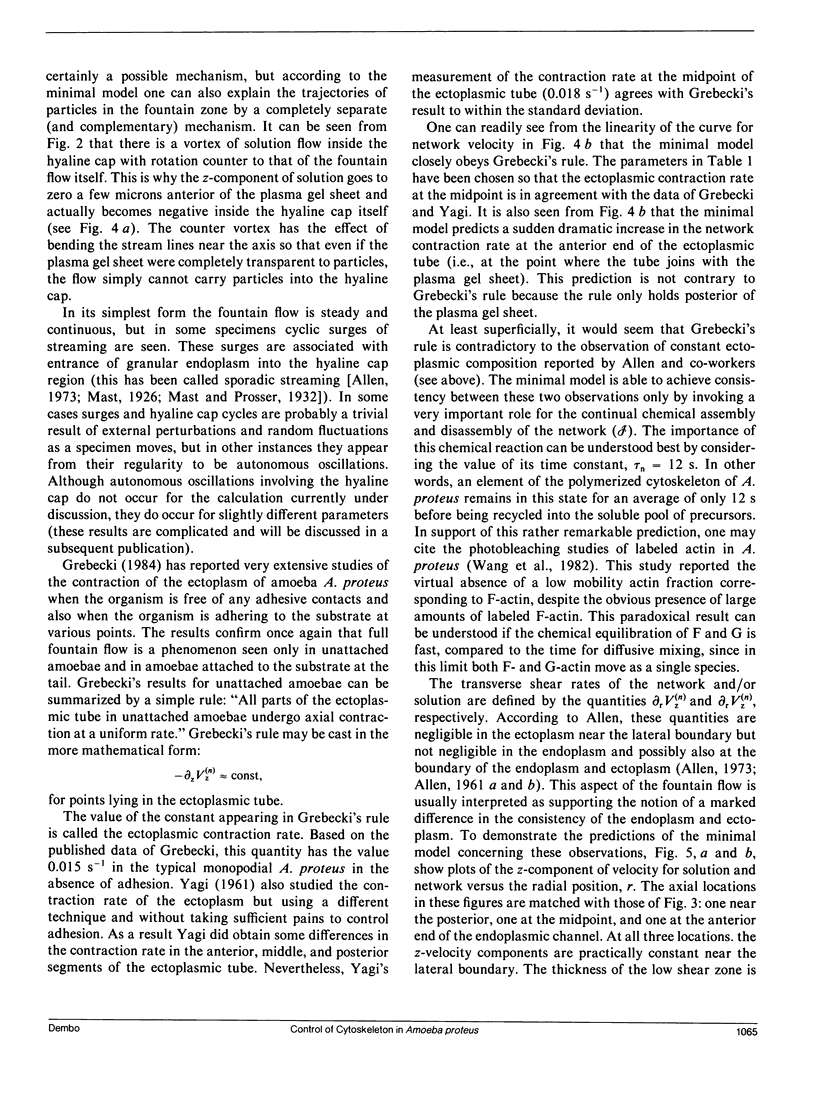
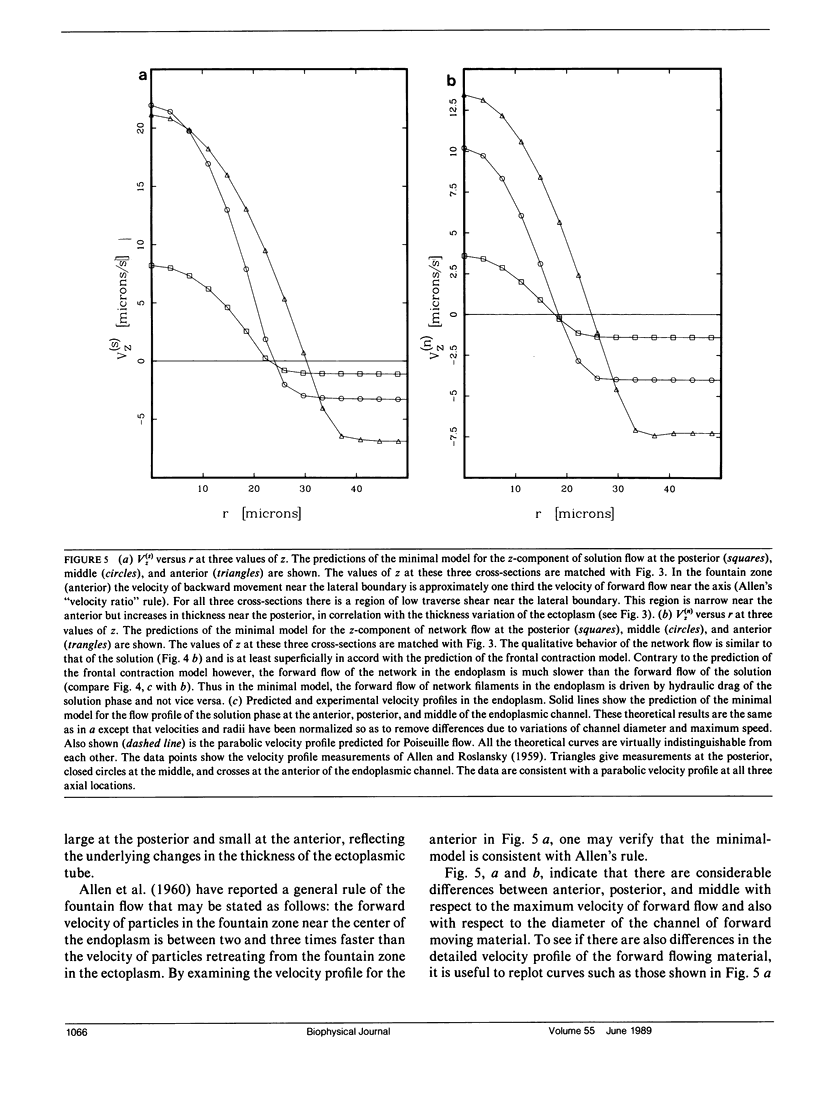
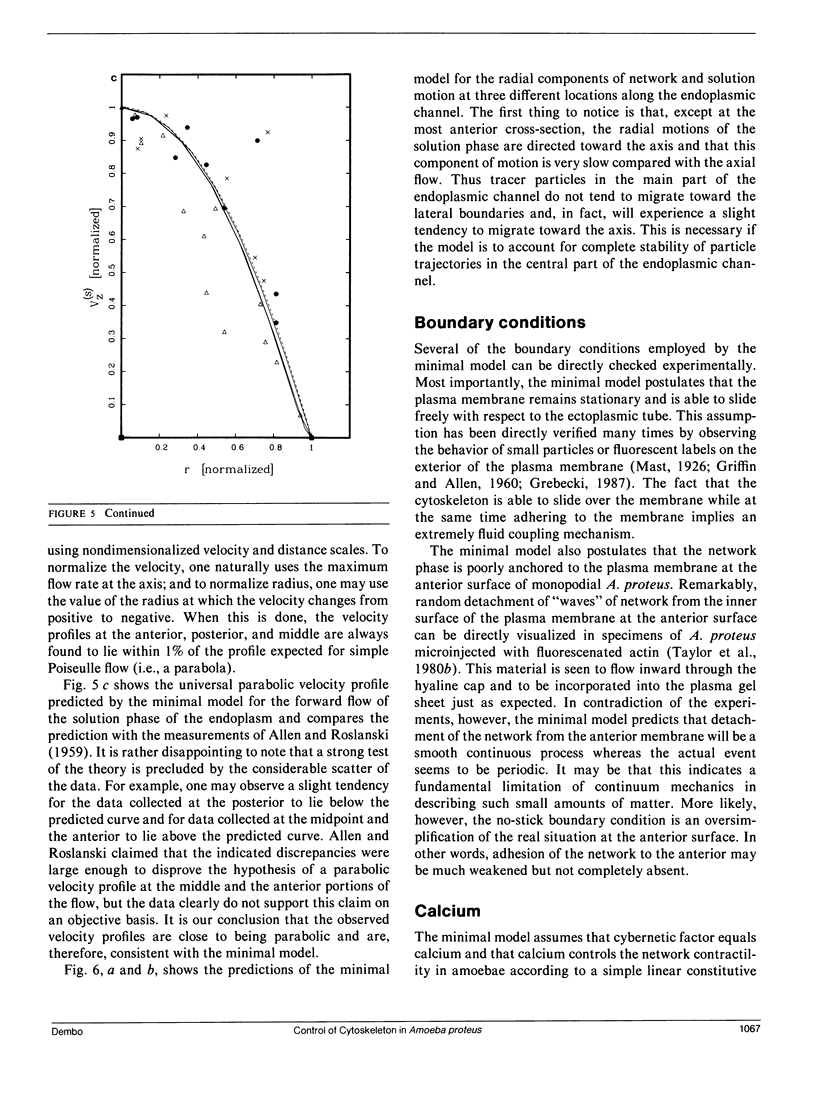
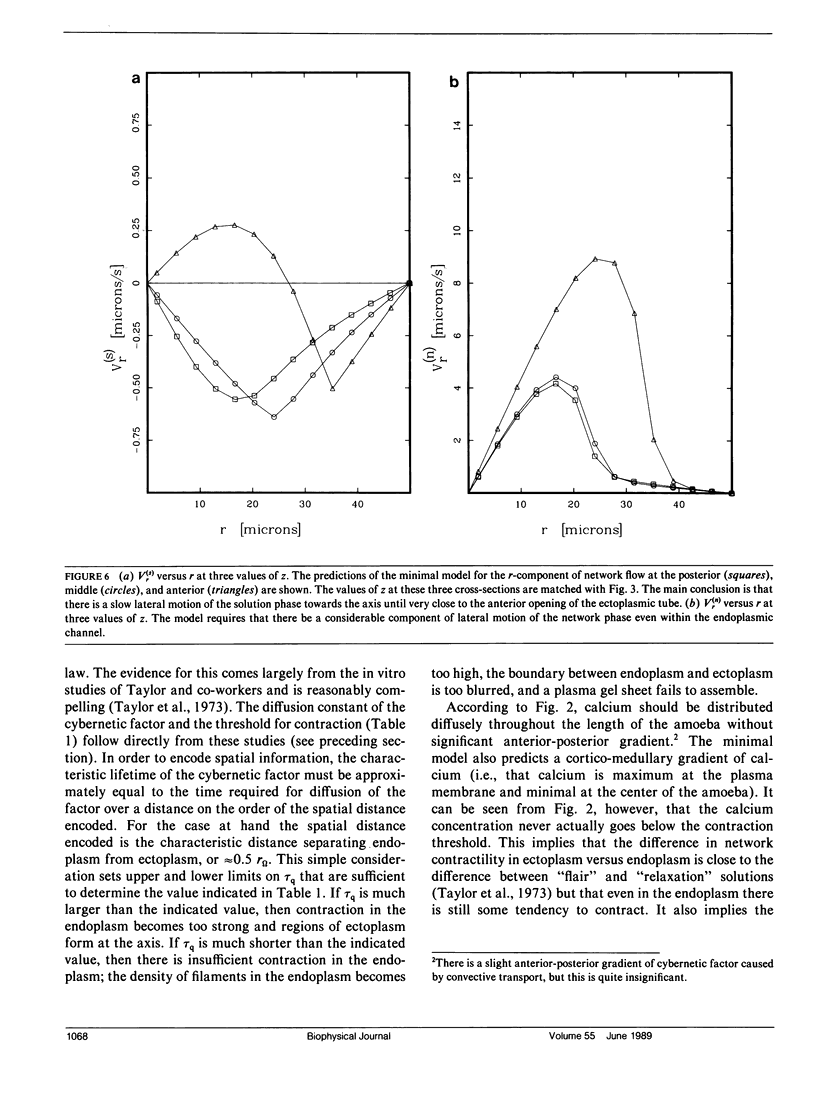
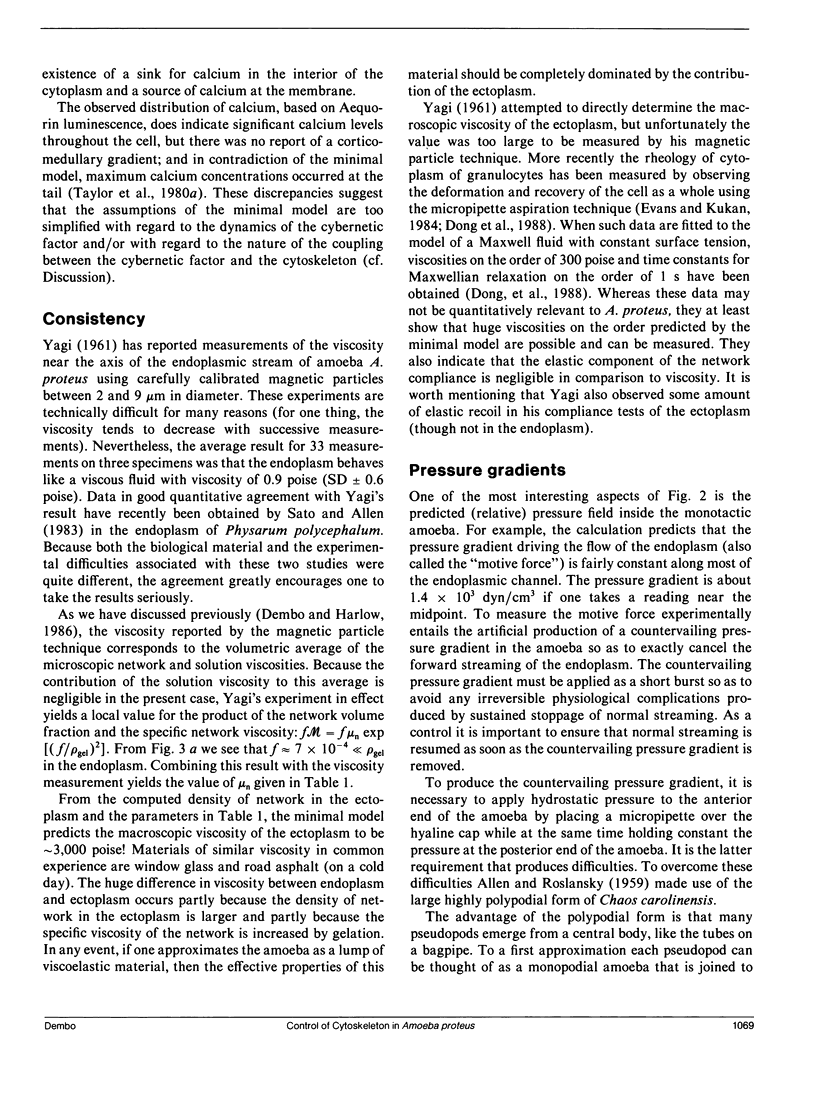
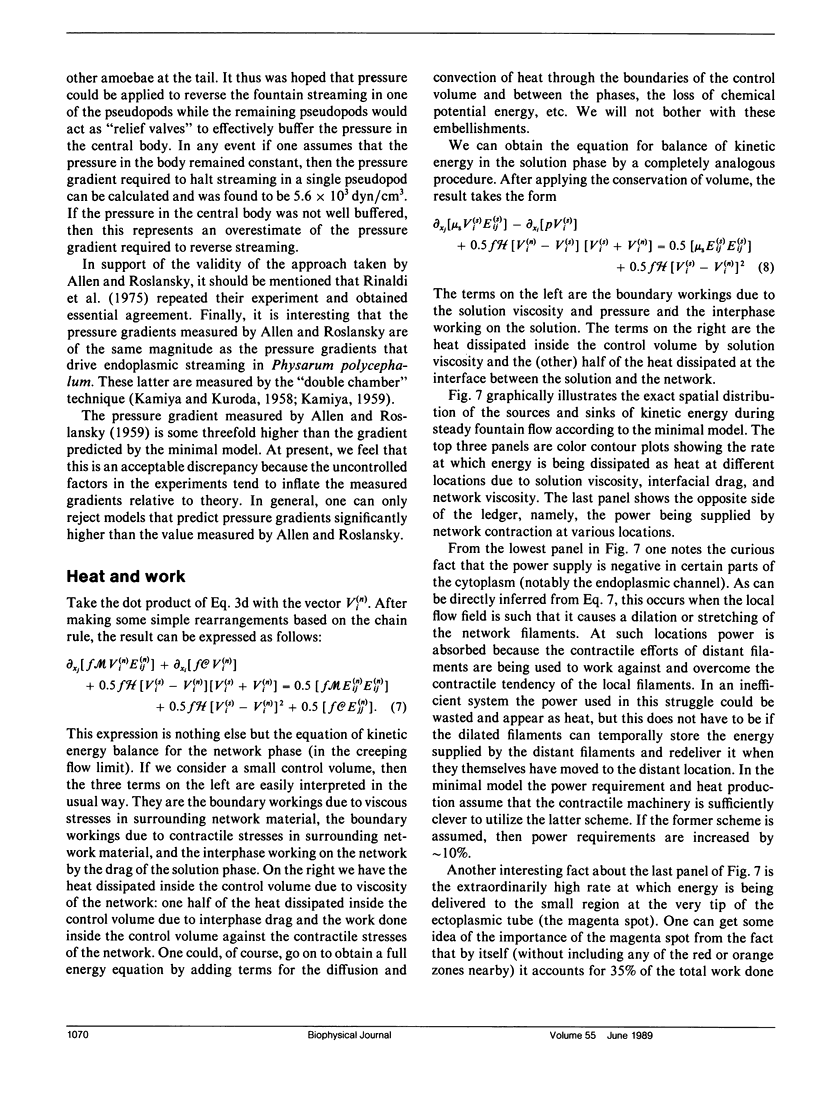
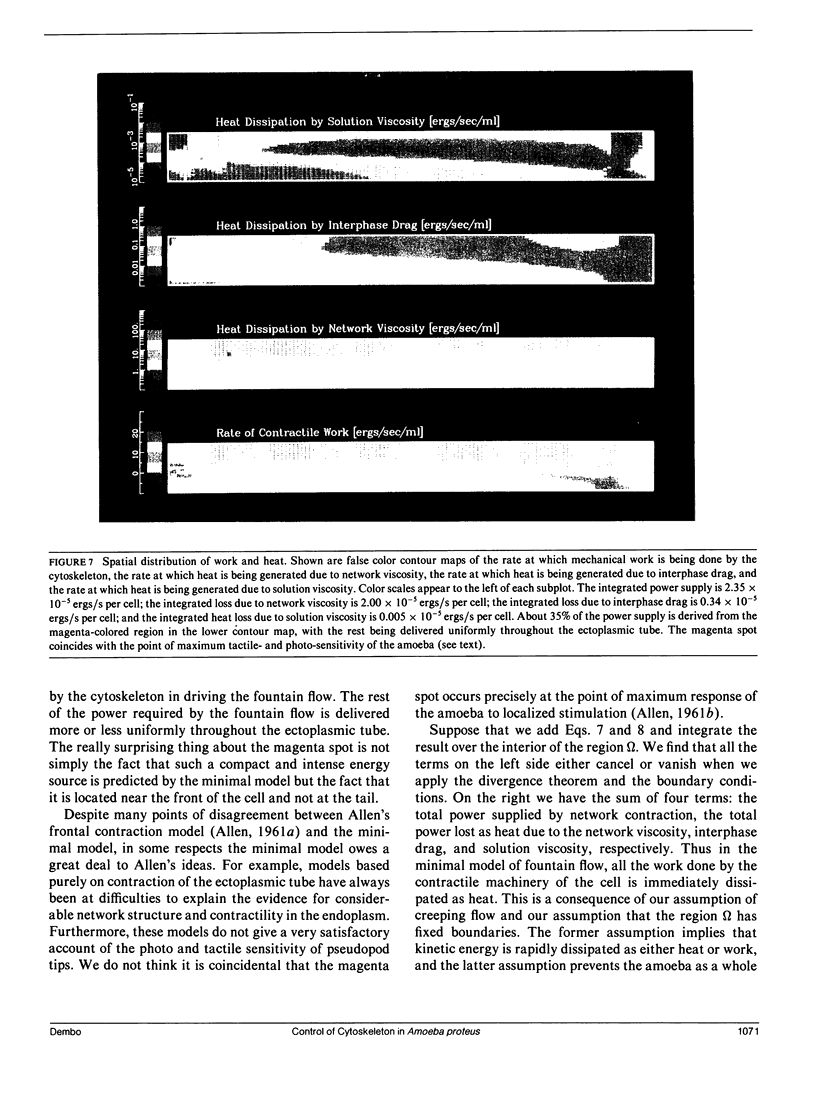
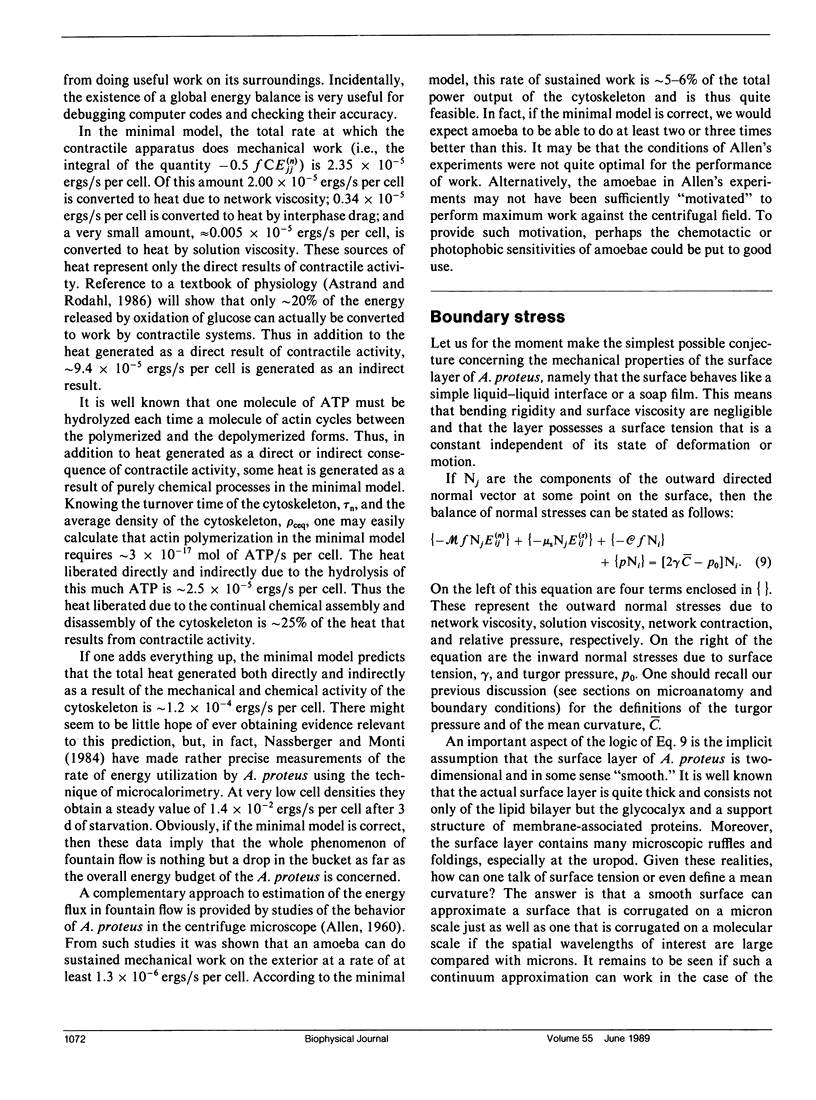
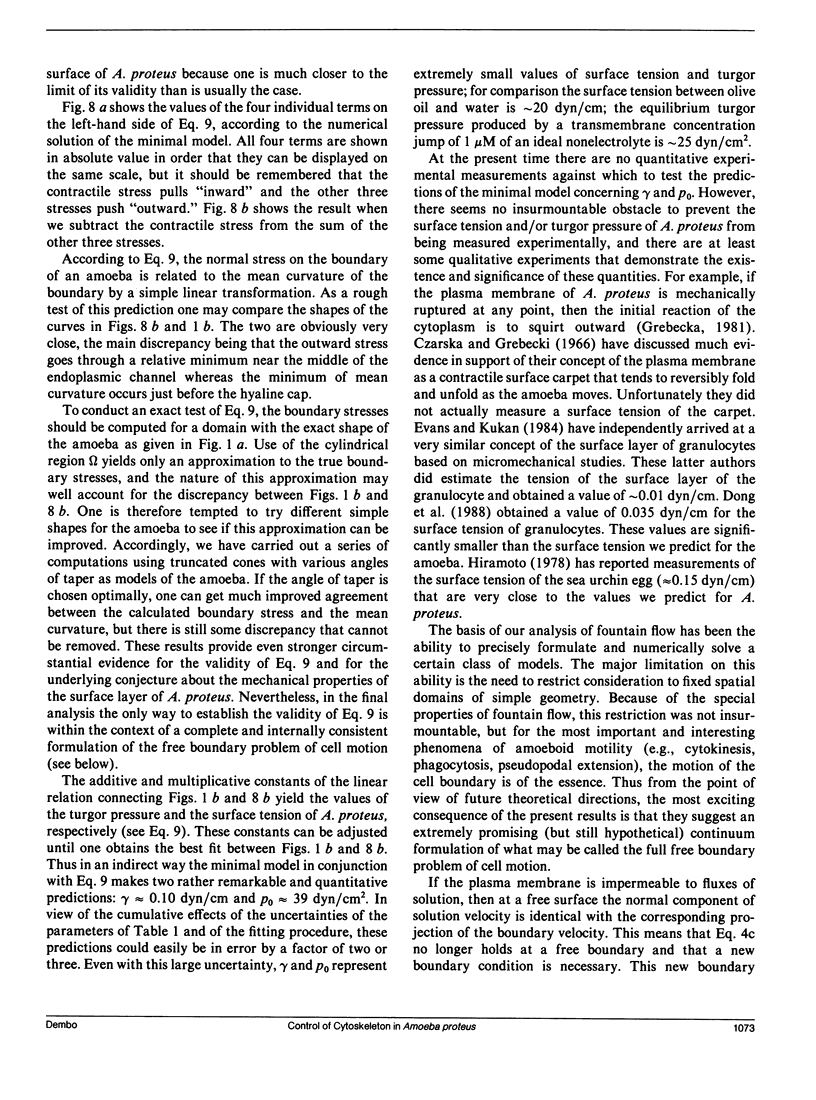
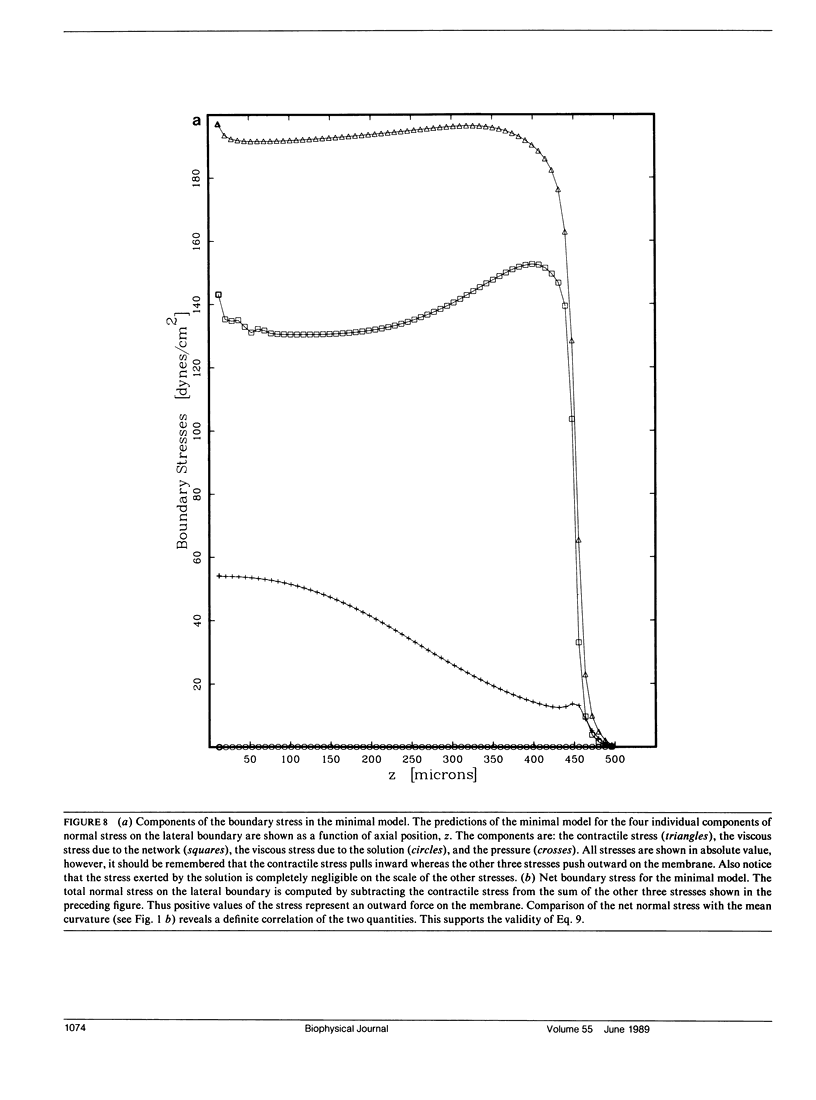
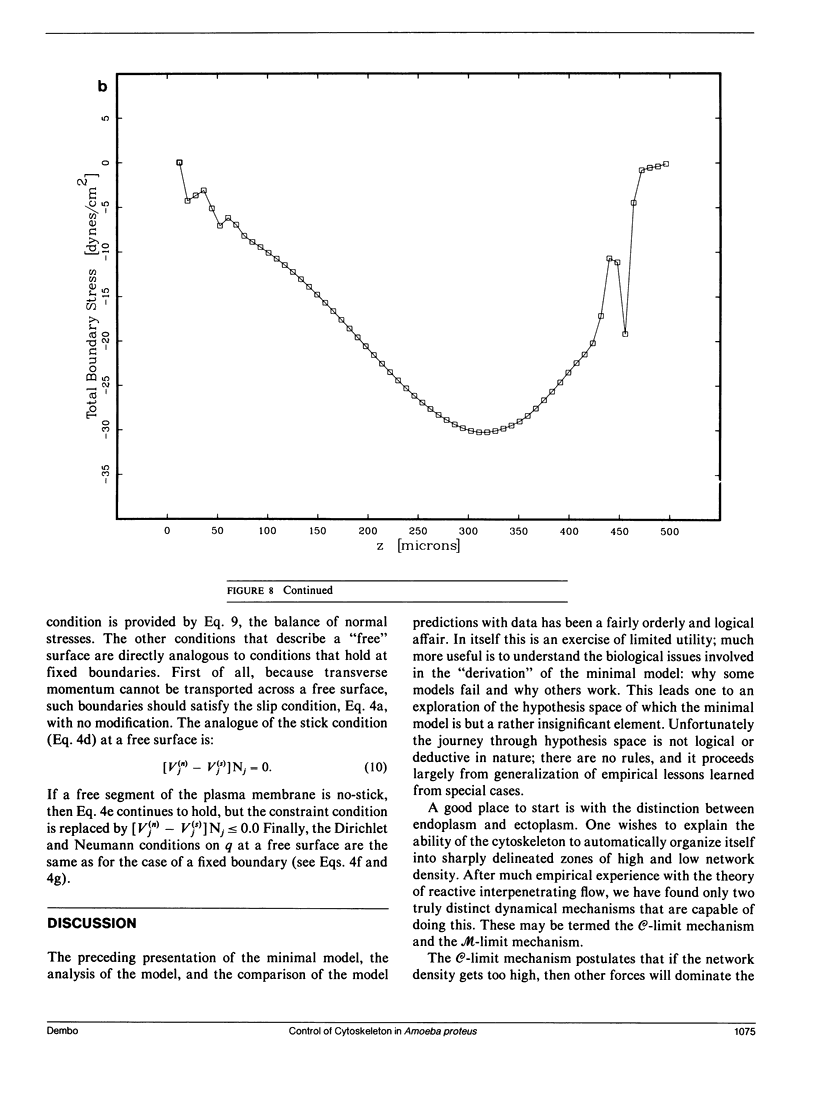
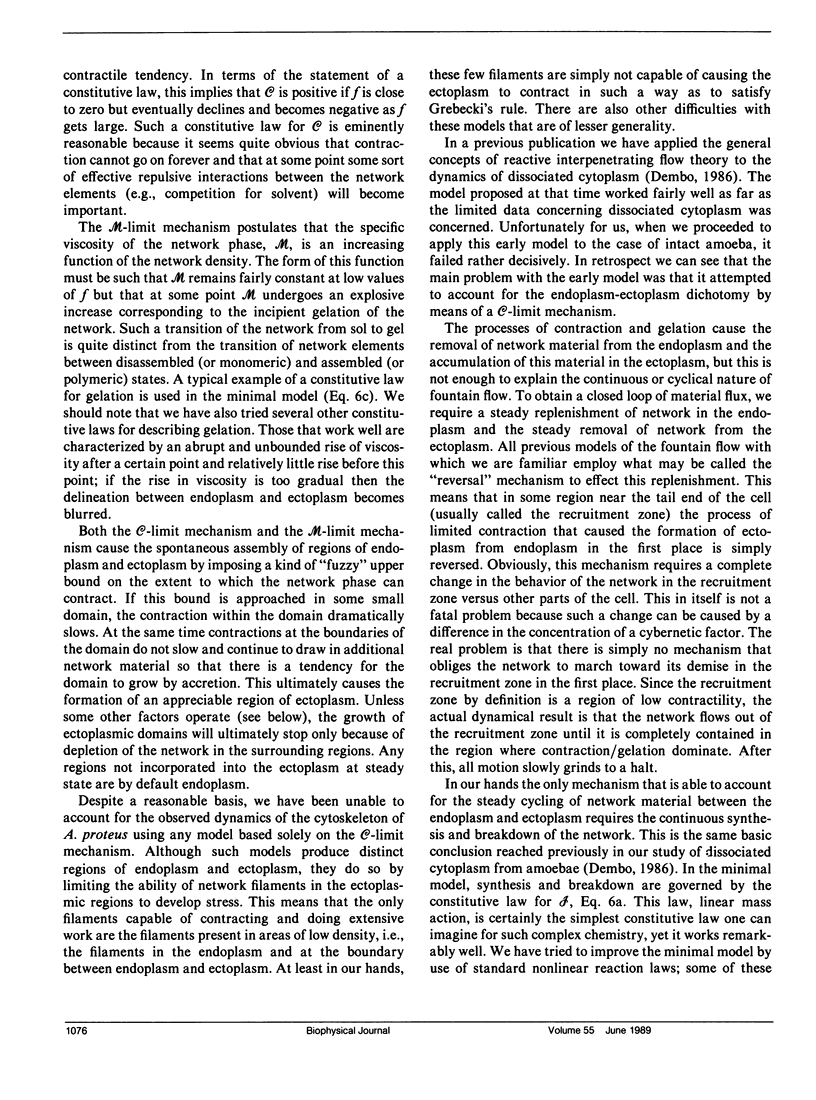

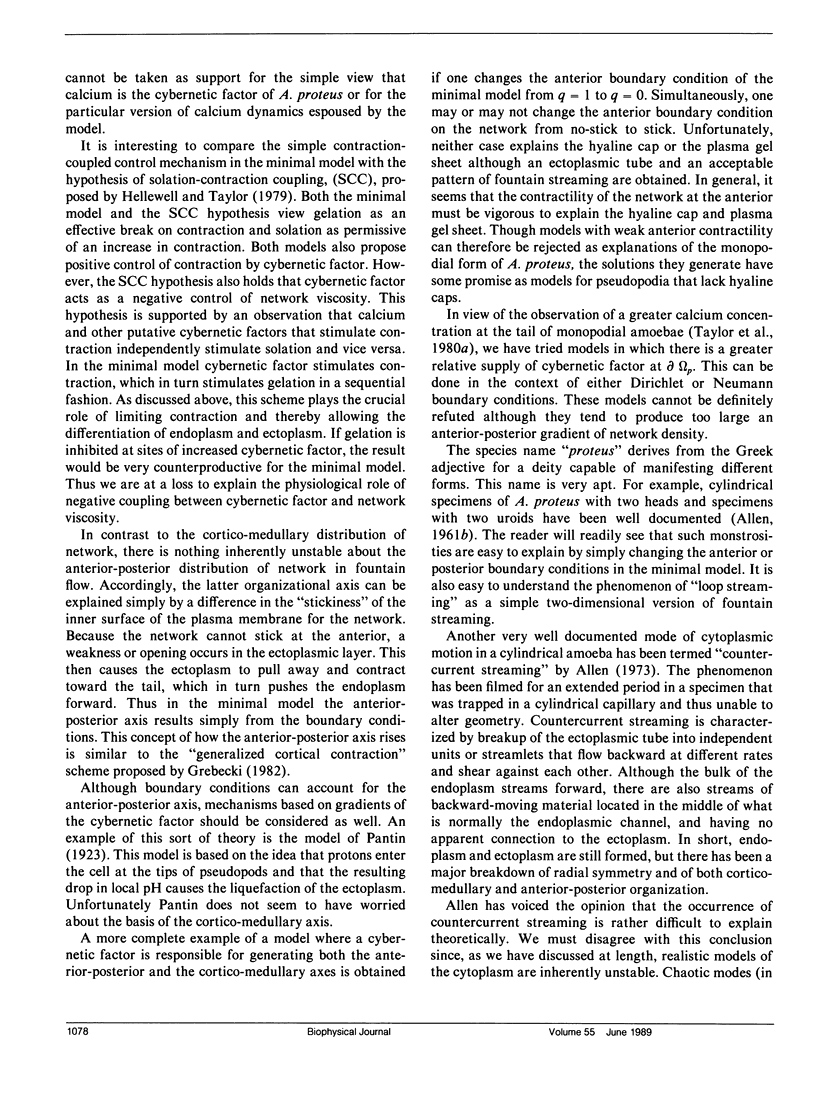
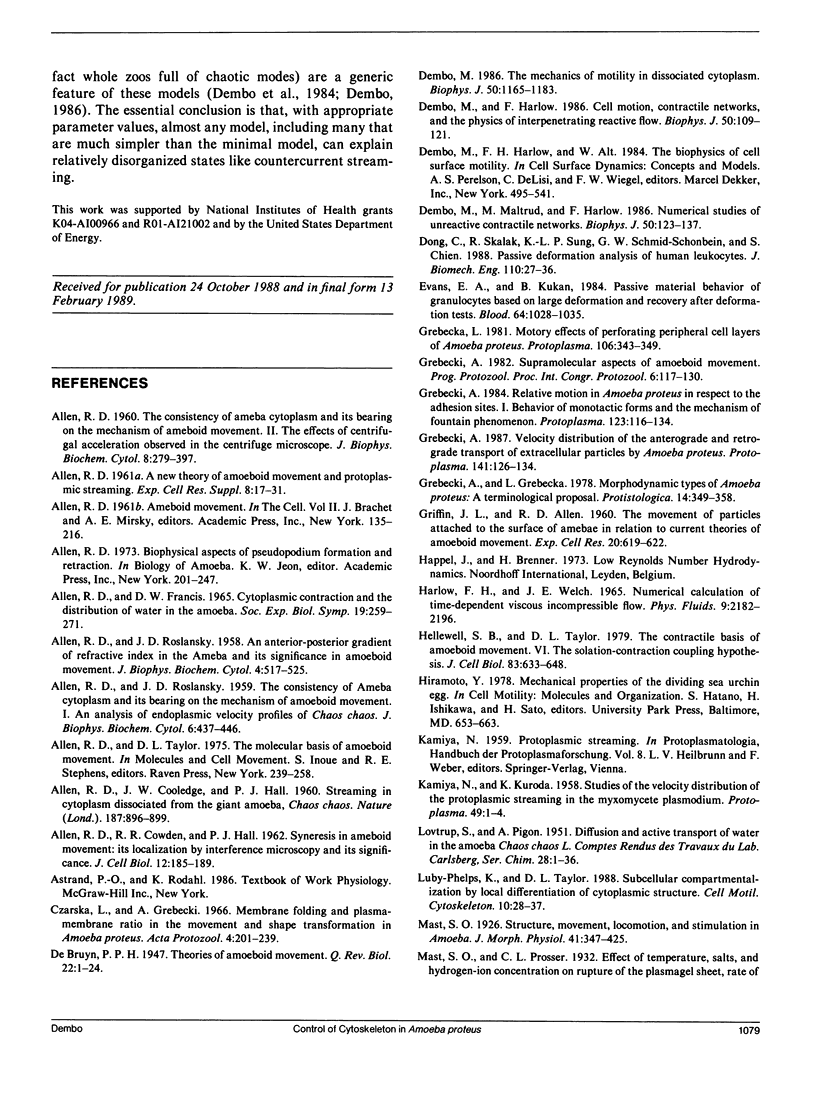
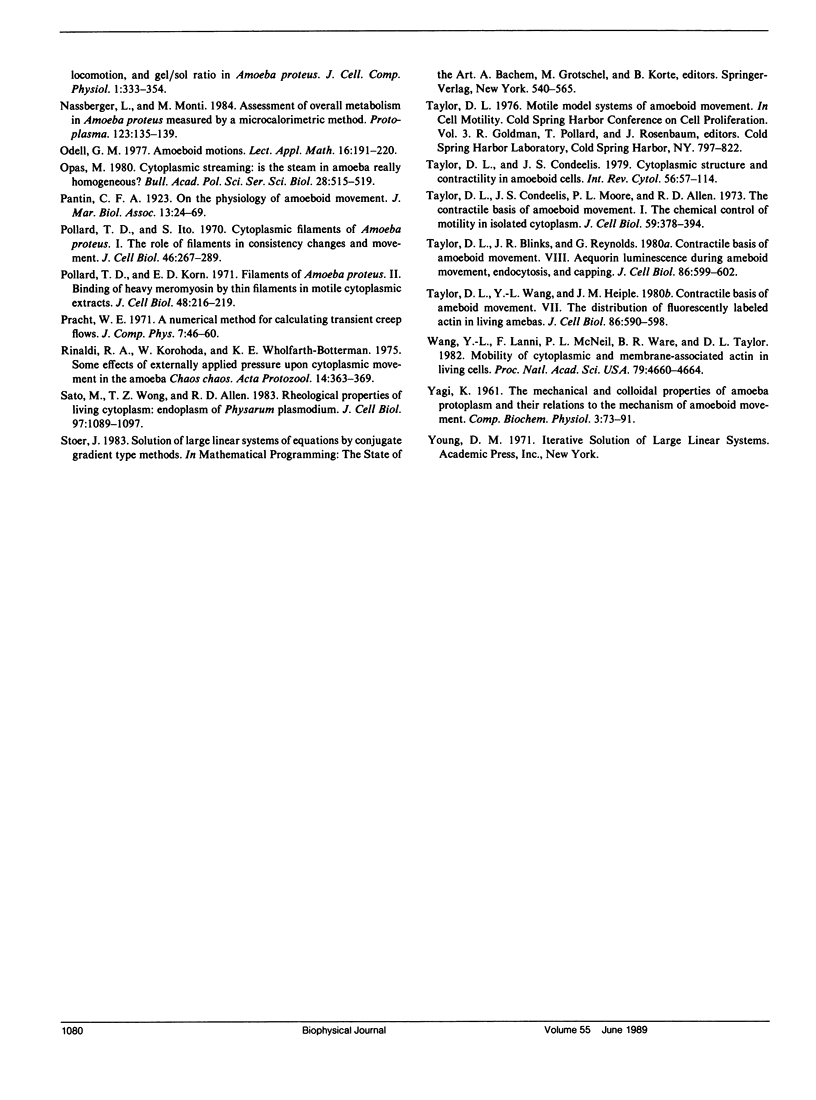
Images in this article
Selected References
These references are in PubMed. This may not be the complete list of references from this article.
- ALLEN R. D. A new theory of ameboid movement and protoplasmic streaming. Exp Cell Res. 1961;Suppl 8:17–31. doi: 10.1016/0014-4827(61)90337-8. [DOI] [PubMed] [Google Scholar]
- ALLEN R. D., COOLEDGE J. W., HALL P. J. Streaming in cytoplasm dissociated from the giant amoeba, Chaos chaos. Nature. 1960 Sep 10;187:896–899. doi: 10.1038/187896a0. [DOI] [PubMed] [Google Scholar]
- ALLEN R. D., COWDEN R. R. Syneresis in ameboid movement: its localization by interference microscopy and its significance. J Cell Biol. 1962 Jan;12:185–189. doi: 10.1083/jcb.12.1.185. [DOI] [PMC free article] [PubMed] [Google Scholar]
- ALLEN R. D., ROSLANSKY J. D. An anterior-posterior gradient of refractive index in the ameba and its significance in ameboid movement. J Biophys Biochem Cytol. 1958 Sep 25;4(5):517–523. doi: 10.1083/jcb.4.5.517. [DOI] [PMC free article] [PubMed] [Google Scholar]
- ALLEN R. D., ROSLANSKY J. D. The consistency of ameba cytoplasm and its bearing on the mechanism of ameboid movement. I. An analysis of endoplasmic velocity profiles of Chaos (L.). J Biophys Biochem Cytol. 1959 Dec;6:437–446. doi: 10.1083/jcb.6.3.437. [DOI] [PMC free article] [PubMed] [Google Scholar]
- ALLEN R. D. The consistency of ameba cytoplasm and its bearing on the mechanism of ameboid movement. II. The effects of centrifugal acceleration observed in the centrifuge microscope. J Biophys Biochem Cytol. 1960 Oct;8:379–397. doi: 10.1083/jcb.8.2.379. [DOI] [PMC free article] [PubMed] [Google Scholar]
- Allen R. D., Francis D. W. Cytoplasmic contraction and the distribution of water in the amoeba. Symp Soc Exp Biol. 1965;19:259–271. [PubMed] [Google Scholar]
- Dembo M., Harlow F. Cell motion, contractile networks, and the physics of interpenetrating reactive flow. Biophys J. 1986 Jul;50(1):109–121. doi: 10.1016/S0006-3495(86)83444-0. [DOI] [PMC free article] [PubMed] [Google Scholar]
- Dembo M., Maltrud M., Harlow F. Numerical studies of unreactive contractile networks. Biophys J. 1986 Jul;50(1):123–137. doi: 10.1016/S0006-3495(86)83445-2. [DOI] [PMC free article] [PubMed] [Google Scholar]
- Dembo M. The mechanics of motility in dissociated cytoplasm. Biophys J. 1986 Dec;50(6):1165–1183. doi: 10.1016/S0006-3495(86)83560-3. [DOI] [PMC free article] [PubMed] [Google Scholar]
- Dong C., Skalak R., Sung K. L., Schmid-Schönbein G. W., Chien S. Passive deformation analysis of human leukocytes. J Biomech Eng. 1988 Feb;110(1):27–36. doi: 10.1115/1.3108402. [DOI] [PubMed] [Google Scholar]
- Evans E., Kukan B. Passive material behavior of granulocytes based on large deformation and recovery after deformation tests. Blood. 1984 Nov;64(5):1028–1035. [PubMed] [Google Scholar]
- GRIFFIN J. L., ALLEN R. D. The movement of particles attached to the surface of amebae in relation to current theories of ameboid movement. Exp Cell Res. 1960 Sep;20:619–622. doi: 10.1016/0014-4827(60)90140-3. [DOI] [PubMed] [Google Scholar]
- Hellewell S. B., Taylor D. L. The contractile basis of ameboid movement. VI. The solation-contraction coupling hypothesis. J Cell Biol. 1979 Dec;83(3):633–648. doi: 10.1083/jcb.83.3.633. [DOI] [PMC free article] [PubMed] [Google Scholar]
- Luby-Phelps K., Taylor D. L. Subcellular compartmentalization by local differentiation of cytoplasmic structure. Cell Motil Cytoskeleton. 1988;10(1-2):28–37. doi: 10.1002/cm.970100107. [DOI] [PubMed] [Google Scholar]
- LØVTRUP S., PIGON A. Diffusion and active transport of water in amoeba Chaos chaos l. C R Trav Lab Carlsberg Chim. 1952;28(1):1–36. [PubMed] [Google Scholar]
- Pollard T. D., Ito S. Cytoplasmic filaments of Amoeba proteus. I. The role of filaments in consistency changes and movement. J Cell Biol. 1970 Aug;46(2):267–289. doi: 10.1083/jcb.46.2.267. [DOI] [PMC free article] [PubMed] [Google Scholar]
- Pollard T. D., Korn E. D. Filaments of Amoeba proteus. II. Binding of heavy meromyosin by thin filaments in motile cytoplasmic extracts. J Cell Biol. 1971 Jan;48(1):216–219. doi: 10.1083/jcb.48.1.216. [DOI] [PMC free article] [PubMed] [Google Scholar]
- Sato M., Wong T. Z., Allen R. D. Rheological properties of living cytoplasm: endoplasm of Physarum plasmodium. J Cell Biol. 1983 Oct;97(4):1089–1097. doi: 10.1083/jcb.97.4.1089. [DOI] [PMC free article] [PubMed] [Google Scholar]
- Taylor D. L., Blinks J. R., Reynolds G. Contractile basis of ameboid movement. VII. Aequorin luminescence during ameboid movement, endocytosis, and capping. J Cell Biol. 1980 Aug;86(2):599–607. doi: 10.1083/jcb.86.2.599. [DOI] [PMC free article] [PubMed] [Google Scholar]
- Taylor D. L., Condeelis J. S. Cytoplasmic structure and contractility in amoeboid cells. Int Rev Cytol. 1979;56:57–144. doi: 10.1016/s0074-7696(08)61821-5. [DOI] [PubMed] [Google Scholar]
- Taylor D. L., Condeelis J. S., Moore P. L., Allen R. D. The contractile basis of amoeboid movement. I. The chemical control of motility in isolated cytoplasm. J Cell Biol. 1973 Nov;59(2 Pt 1):378–394. doi: 10.1083/jcb.59.2.378. [DOI] [PMC free article] [PubMed] [Google Scholar]
- Taylor D. L., Wang Y. L., Heiple J. M. Contractile basis of ameboid movement. VII. The distribution of fluorescently labeled actin in living amebas. J Cell Biol. 1980 Aug;86(2):590–598. doi: 10.1083/jcb.86.2.590. [DOI] [PMC free article] [PubMed] [Google Scholar]
- Wang Y. L., Lanni F., McNeil P. L., Ware B. R., Taylor D. L. Mobility of cytoplasmic and membrane-associated actin in living cells. Proc Natl Acad Sci U S A. 1982 Aug;79(15):4660–4664. doi: 10.1073/pnas.79.15.4660. [DOI] [PMC free article] [PubMed] [Google Scholar]
- YAGI K. The mechanical and colloidal properties of Amoeba protoplasm and their relations to the mechanism of amoeboid movement. Comp Biochem Physiol. 1961 Aug;3:73–91. doi: 10.1016/0010-406x(61)90134-7. [DOI] [PubMed] [Google Scholar]