Abstract
The Cl-depleted smooth muscle cells of guinea-pig vas deferens rapidly restore their intracellular Cl activity ( aiCl ) to a level 5 times higher than that predicted by a passive distribution when Cl- ions are readmitted to the extracellular solution. Cl reaccumulation, measured using Cl-sensitive micro-electrodes and the uptake of 36Cl, was slowed about 3-fold by the nominal absence of CO2 and HCO3 and about 10-fold by the presence of the anion exchange inhibitor DIDS (4-4'-diisothiocyanostilbene-2,2'-disulphonic acid). However, the usual level of intracellular Cl was eventually attained and neither condition reduced intracellular Cl in normal tissues. The loss of intracellular Cl that occurs when Cl- ions are removed from the extracellular solution was slowed about 3-fold by the nominal absence of CO2 and HCO3 and was accelerated by their readdition. DIDS slowed the fall in aiCl about 10-fold and reduced 36Cl efflux. Intracellular pH (pHi), measured using pH-sensitive micro-electrodes, increased by a mean of 0.73 units when Cl was removed from the superfusing solution in the presence of CO2 and HCO3, and rapidly decreased when Cl was readmitted. These changes are equivalent to an intracellular accumulation and loss of HCO3- ions respectively. The net transmembrane movement of HCO3- ions which would have caused these changes in pHi was calculated using the measured intracellular buffering power ( Aickin , 1984). 25% fewer HCO3- ions than Cl- ions were accumulated and lost and the HCO3 movement was completed 2-3 times faster than the simultaneous Cl movement under the same conditions. The changes in pHi induced by alteration of extracellular Cl were reduced by the nominal absence of CO2 and HCO3 and abolished by the presence of DIDS. The acidification recorded on readmission of Cl in the nominal absence of CO2 and HCO3 was compatible with a metabolic production of about 0.1% CO2. We conclude that Cl-HCO3 exchange plays a major role in the regulation of intracellular Cl. The exchange carrier is reversible, is completely inhibited by DIDS, and accounts for about 75% of the net Cl movement that occurs when Cl is removed from or readmitted to the extracellular solution. The mechanism which is responsible for the remaining Cl movement remains to be elucidated.
Full text
PDF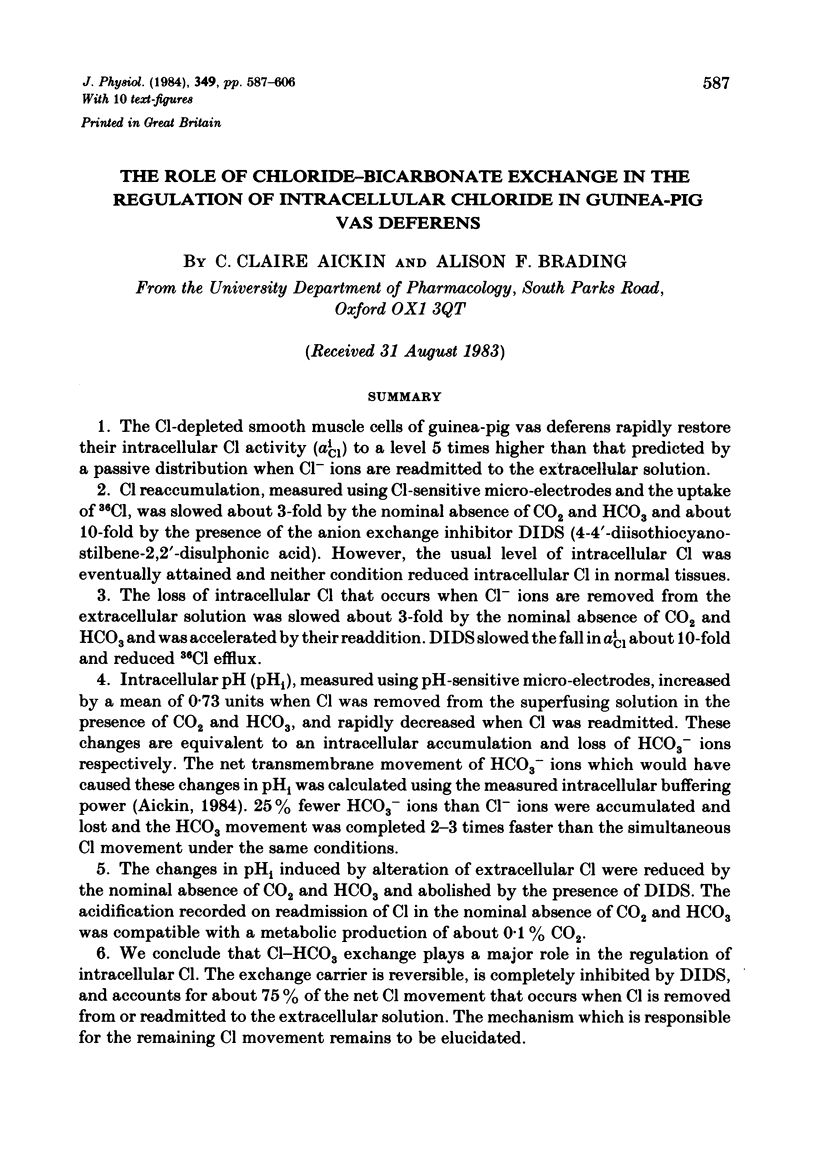
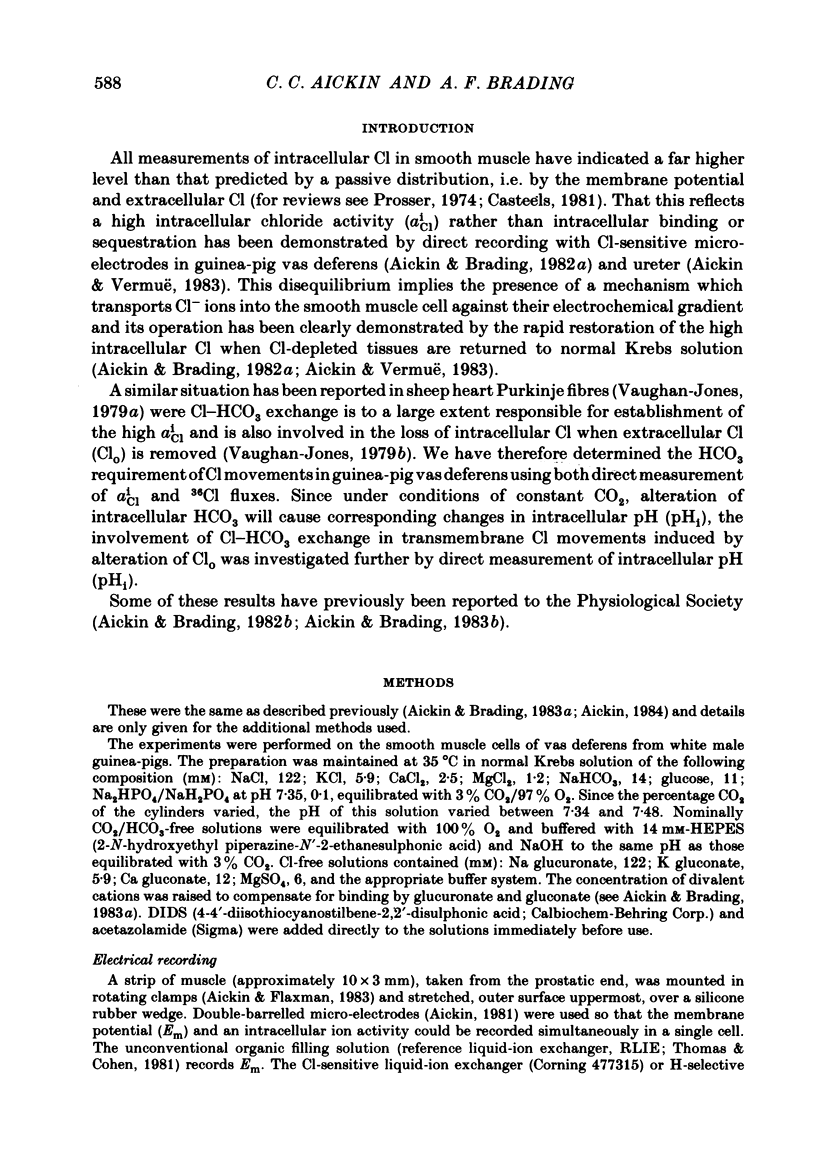
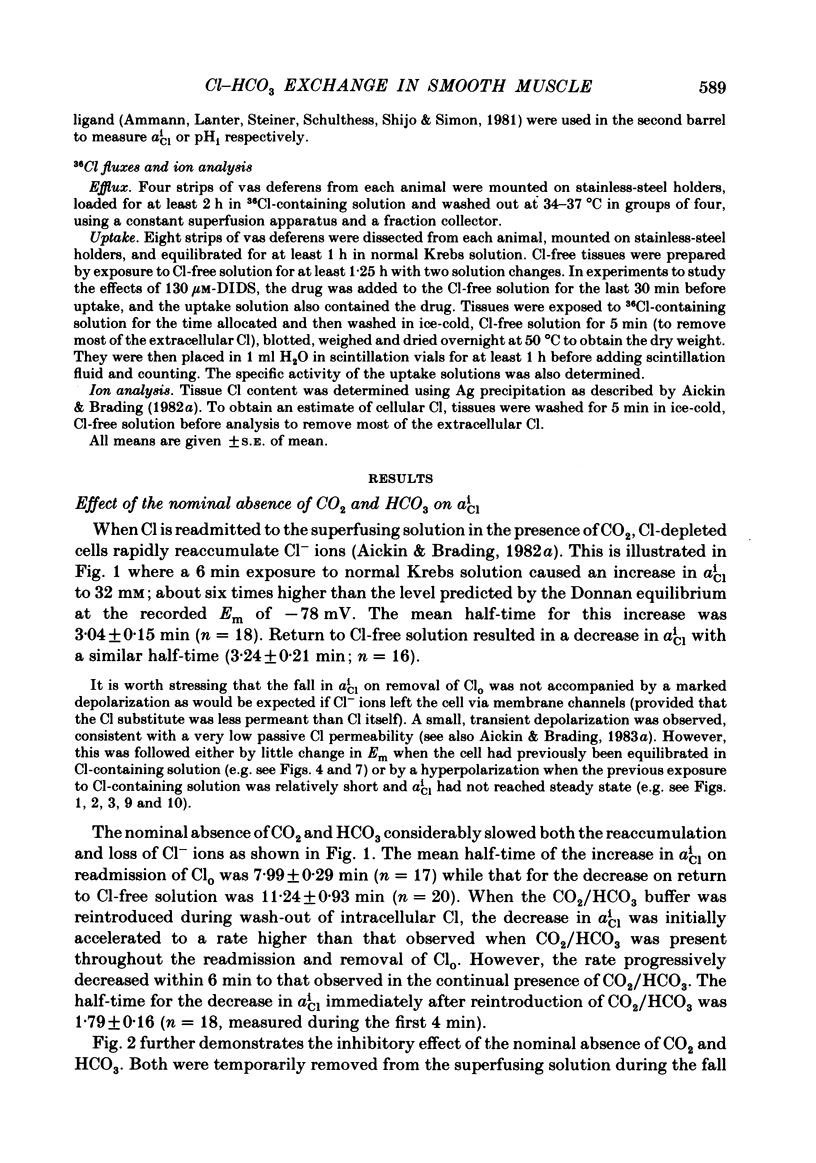
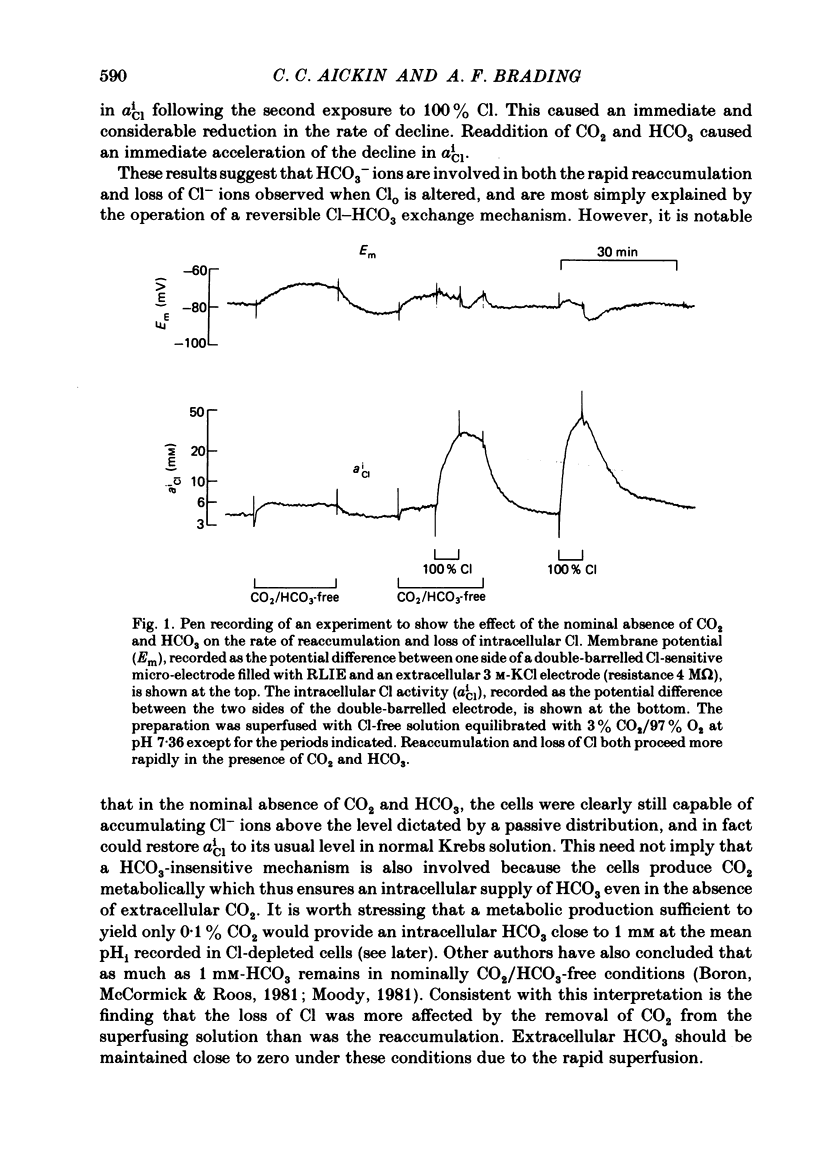
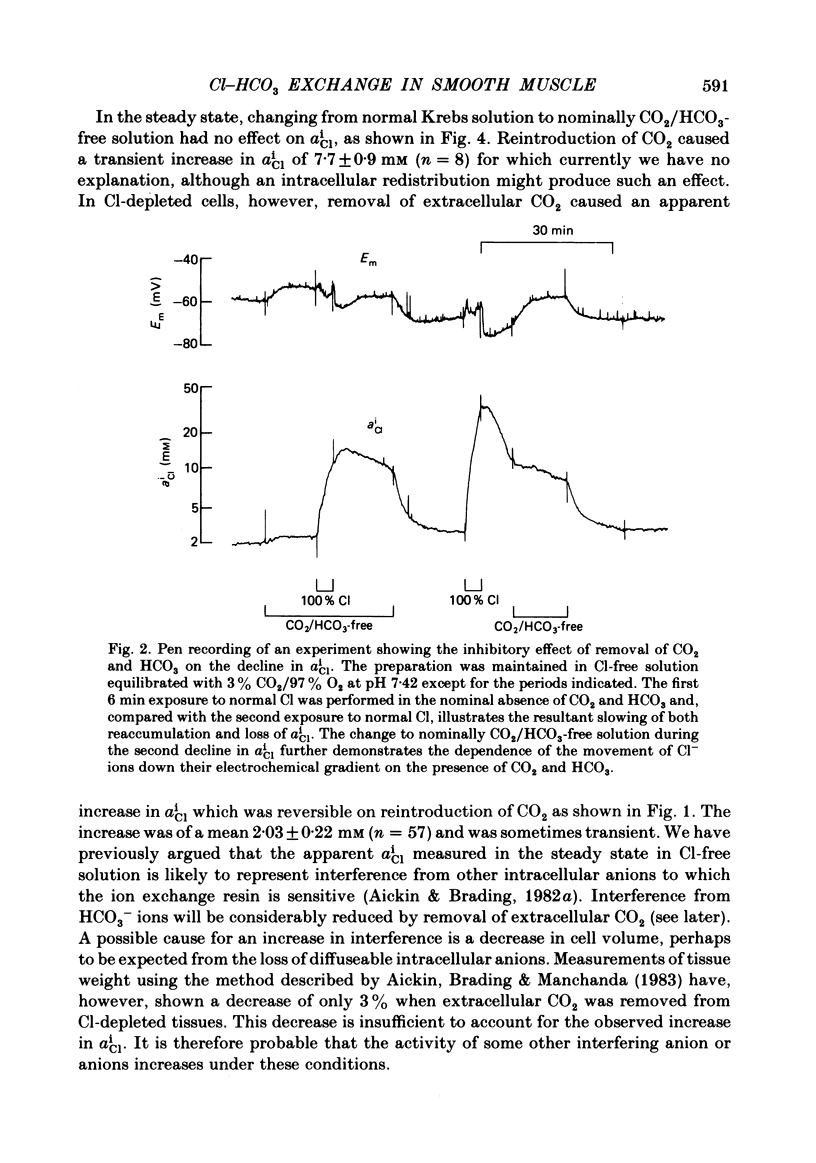
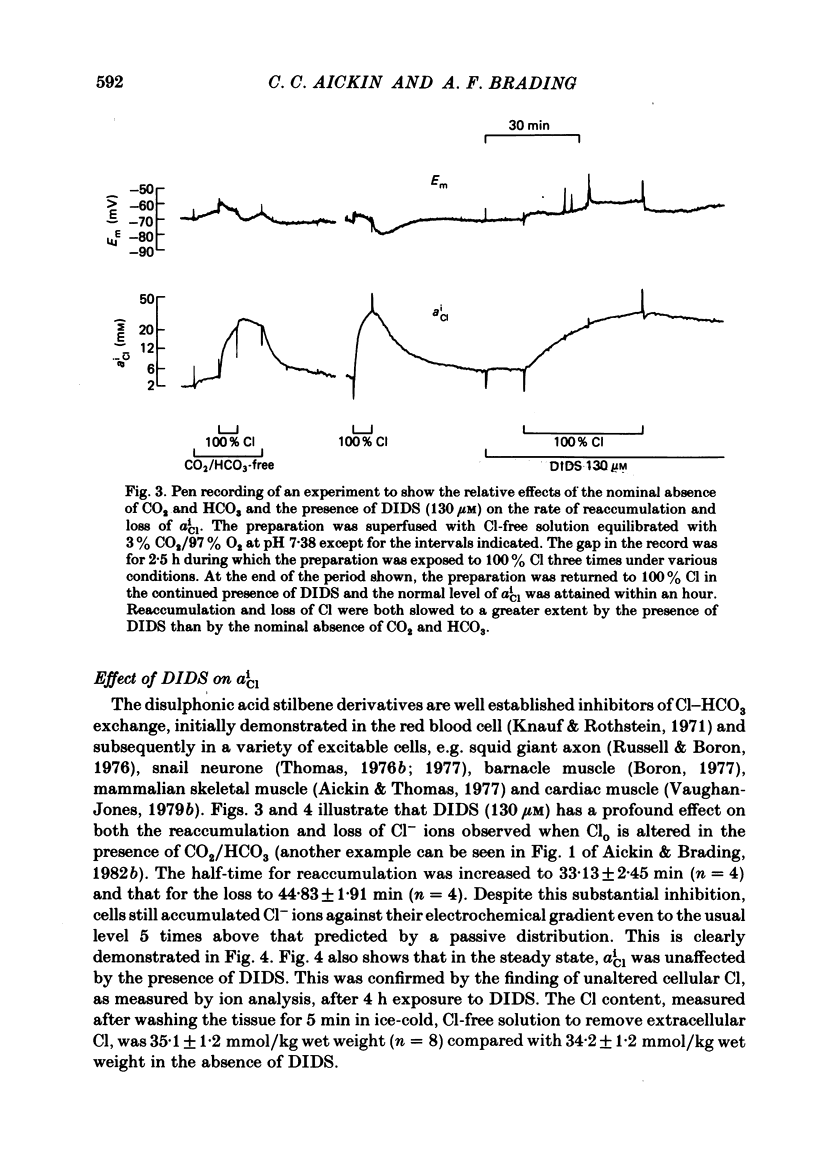
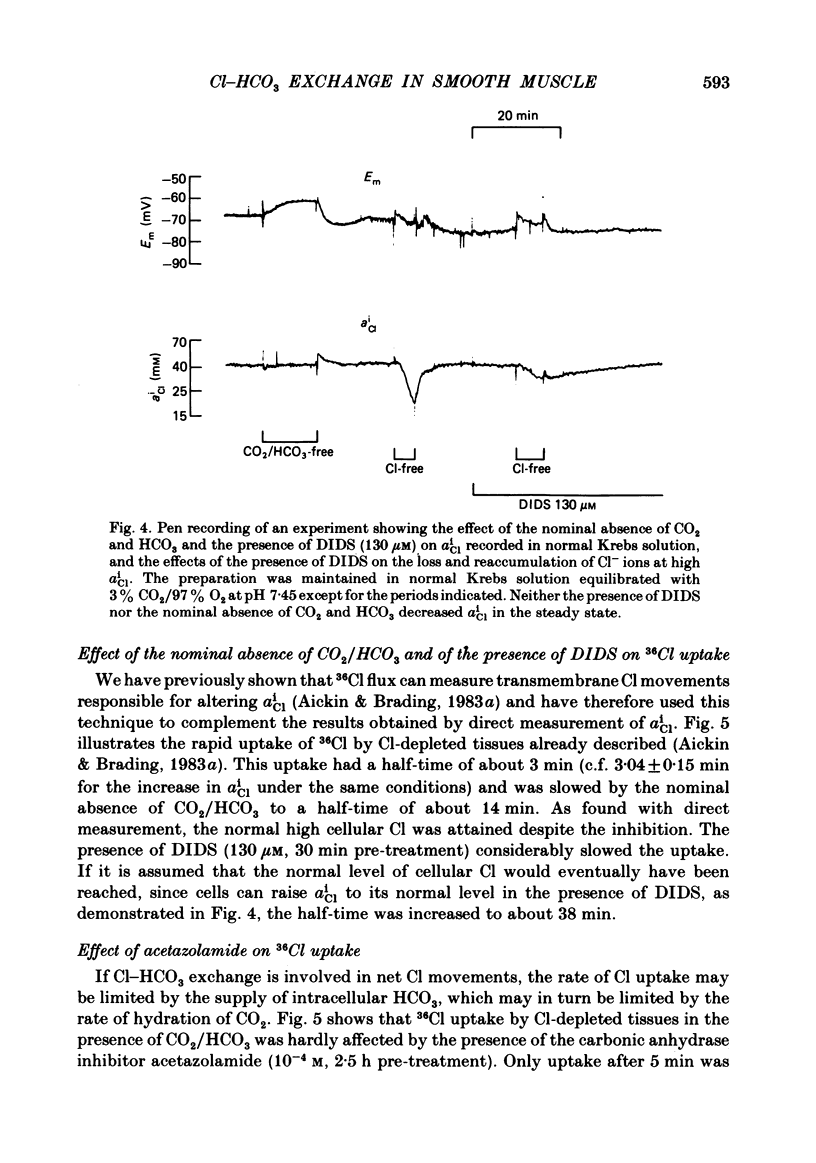
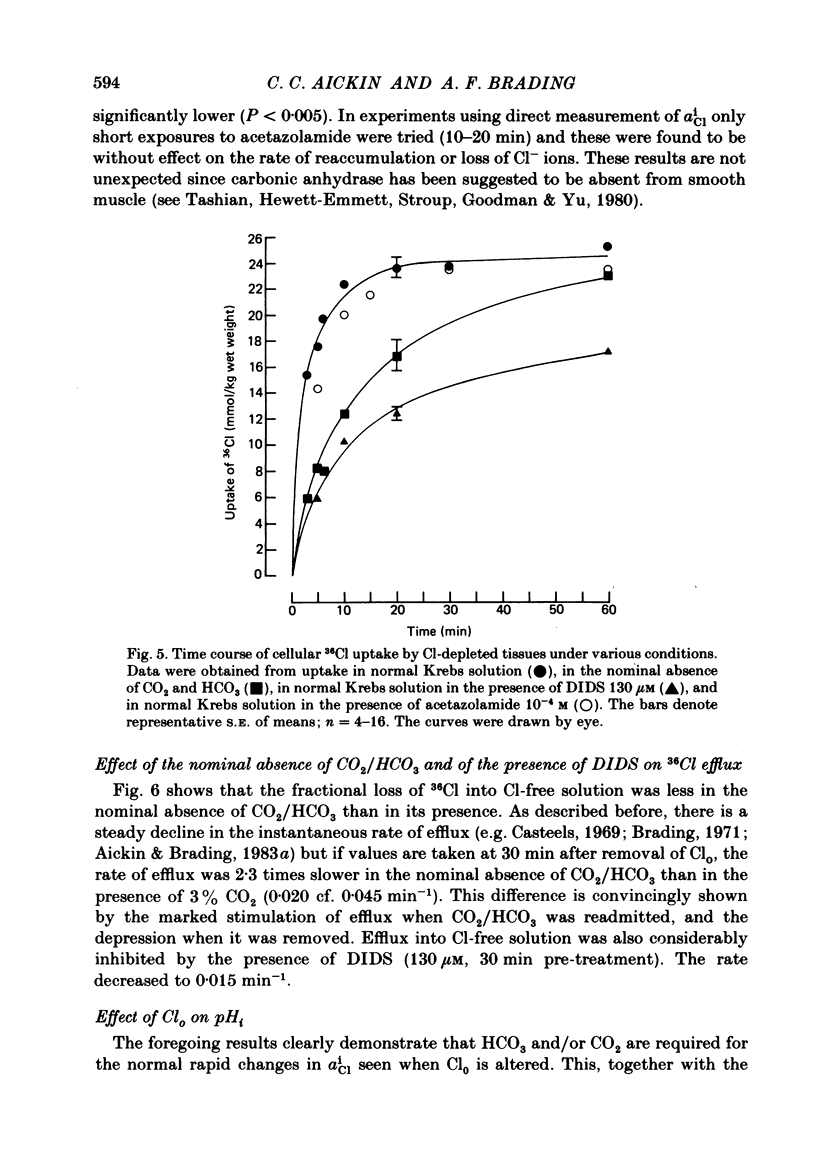
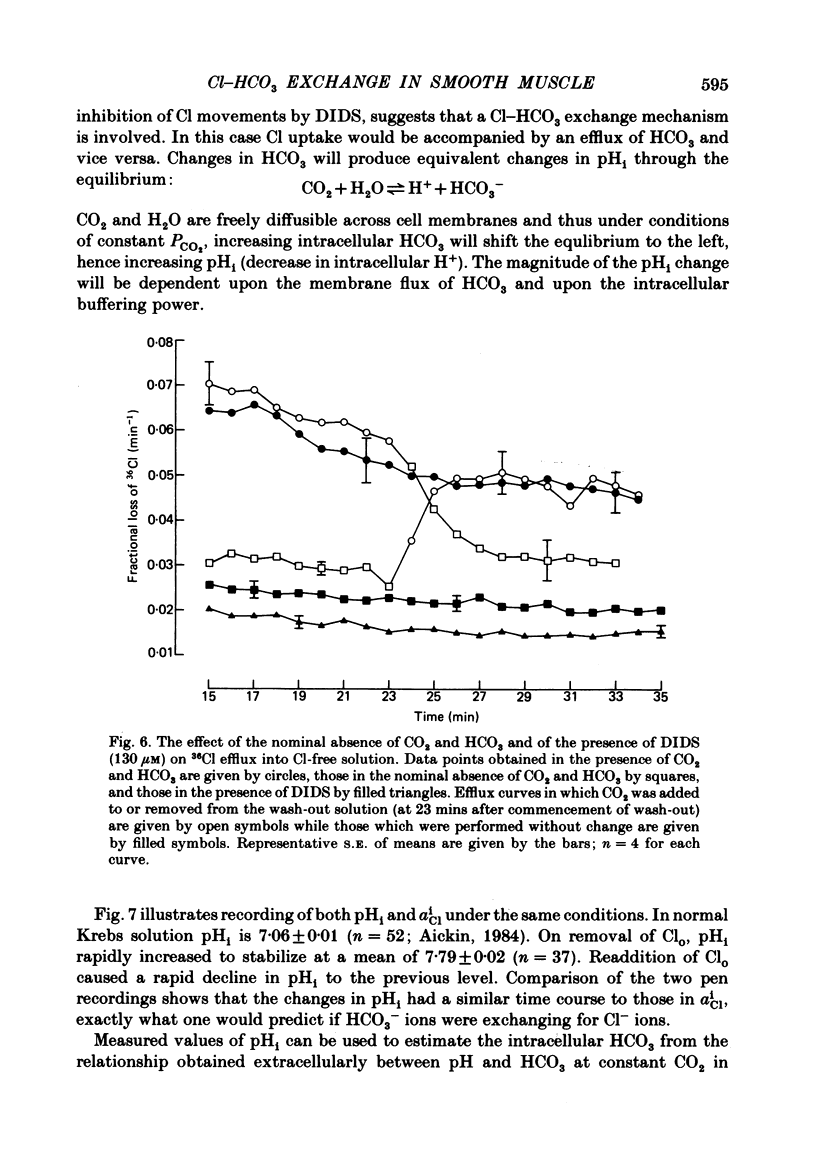
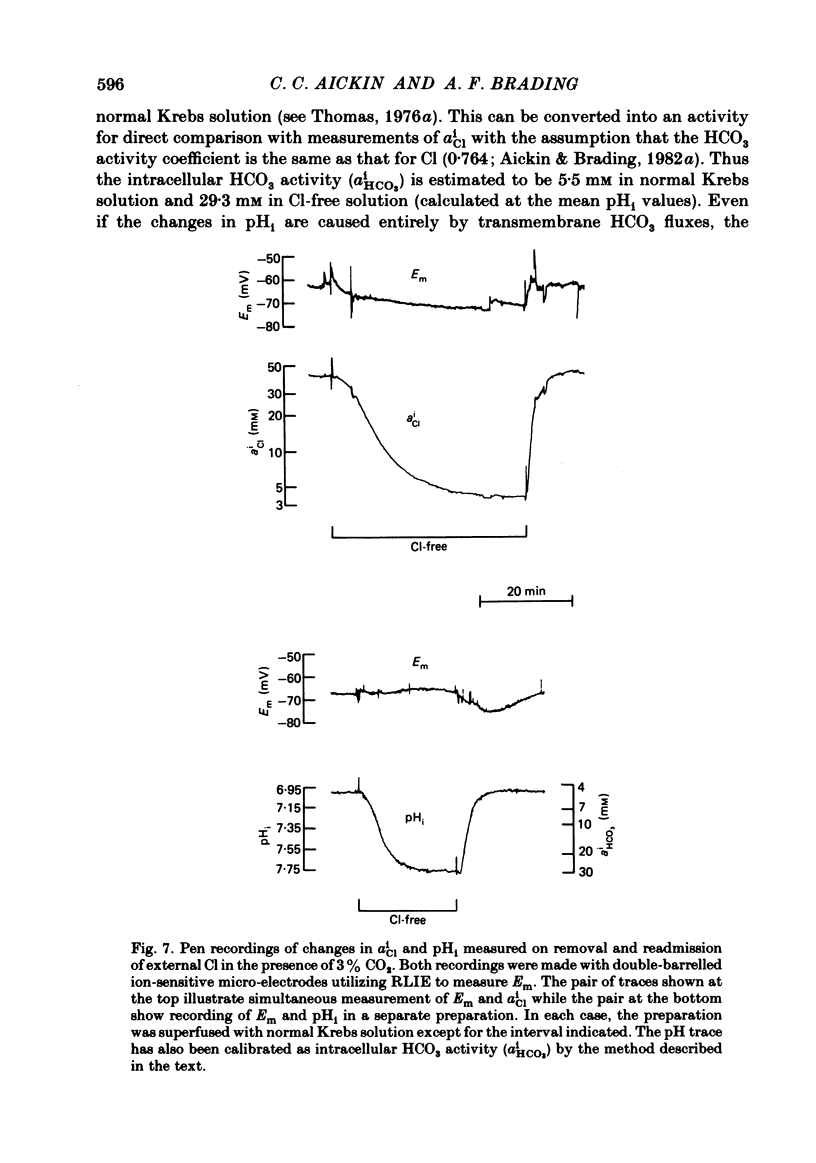
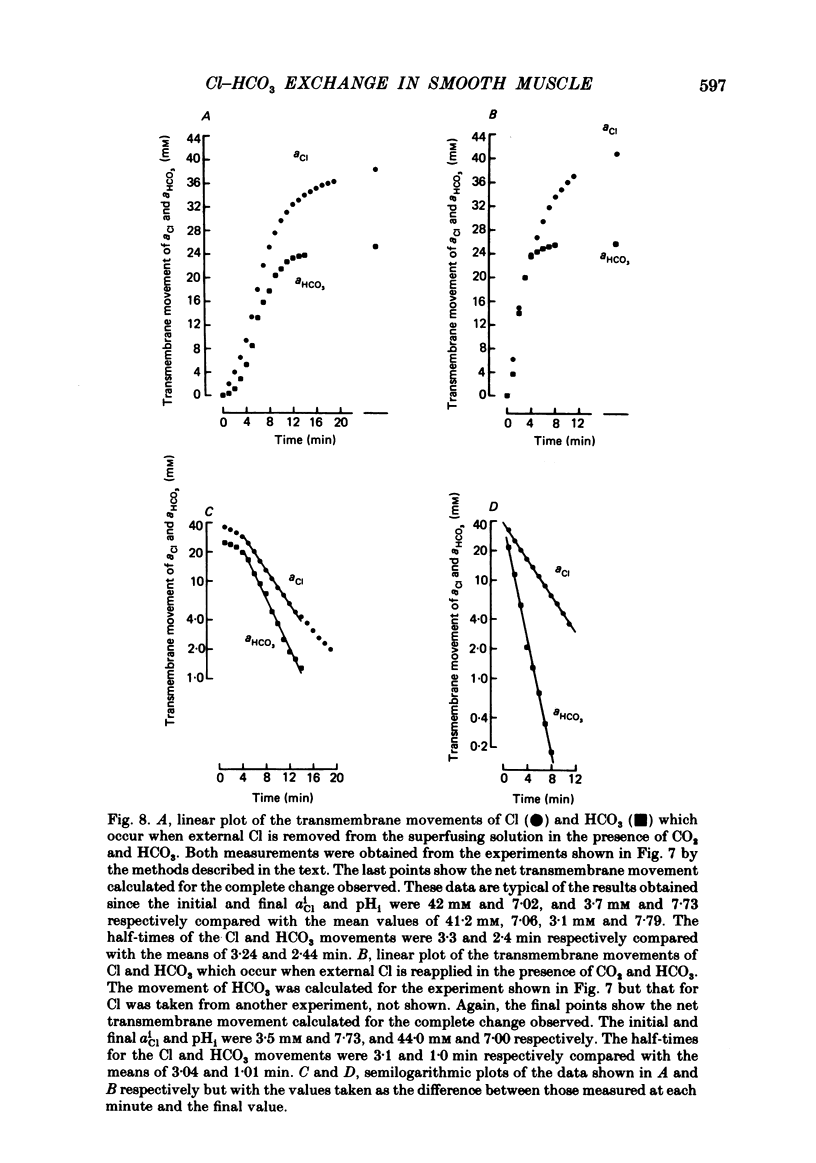
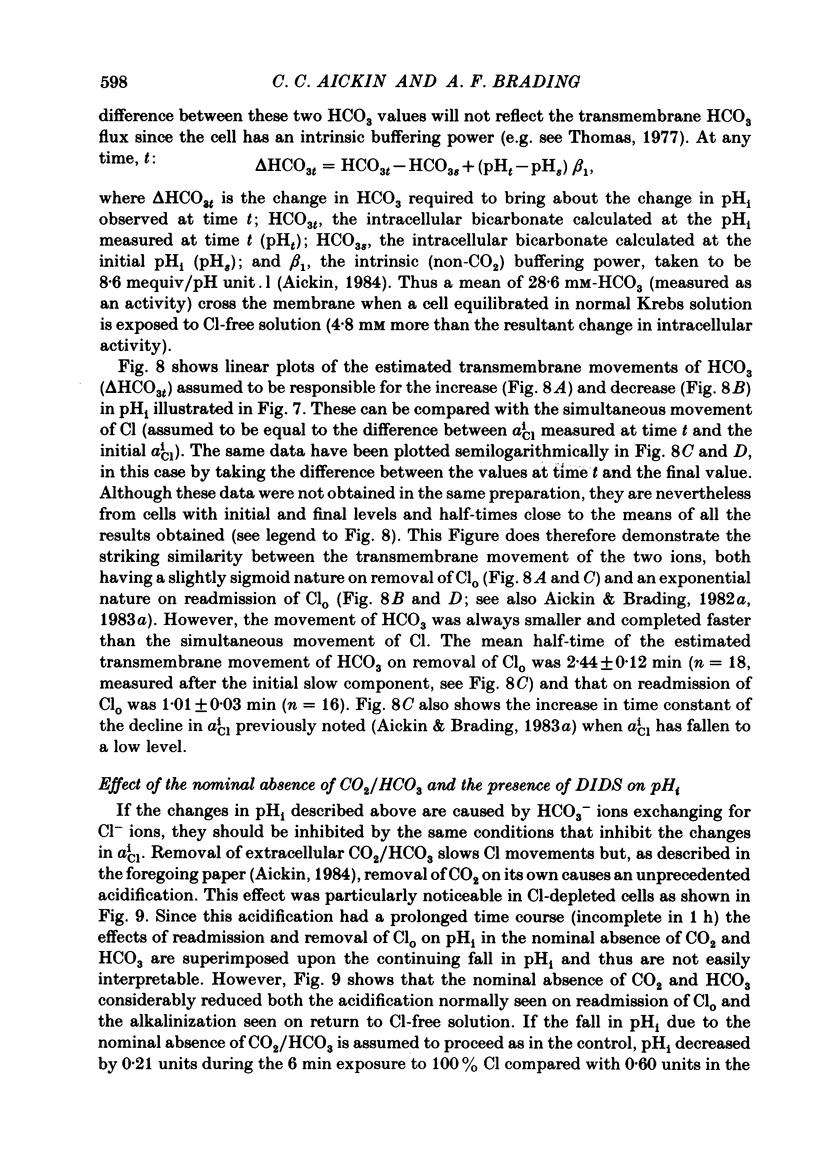
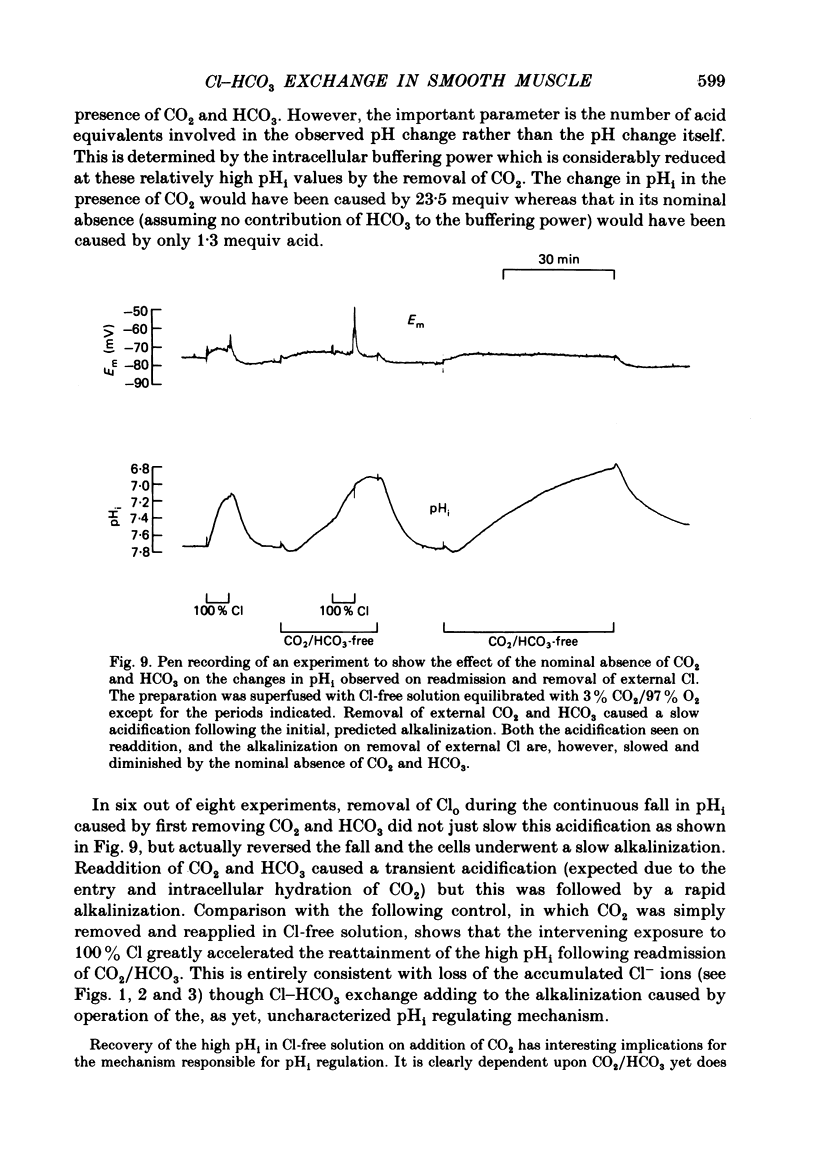
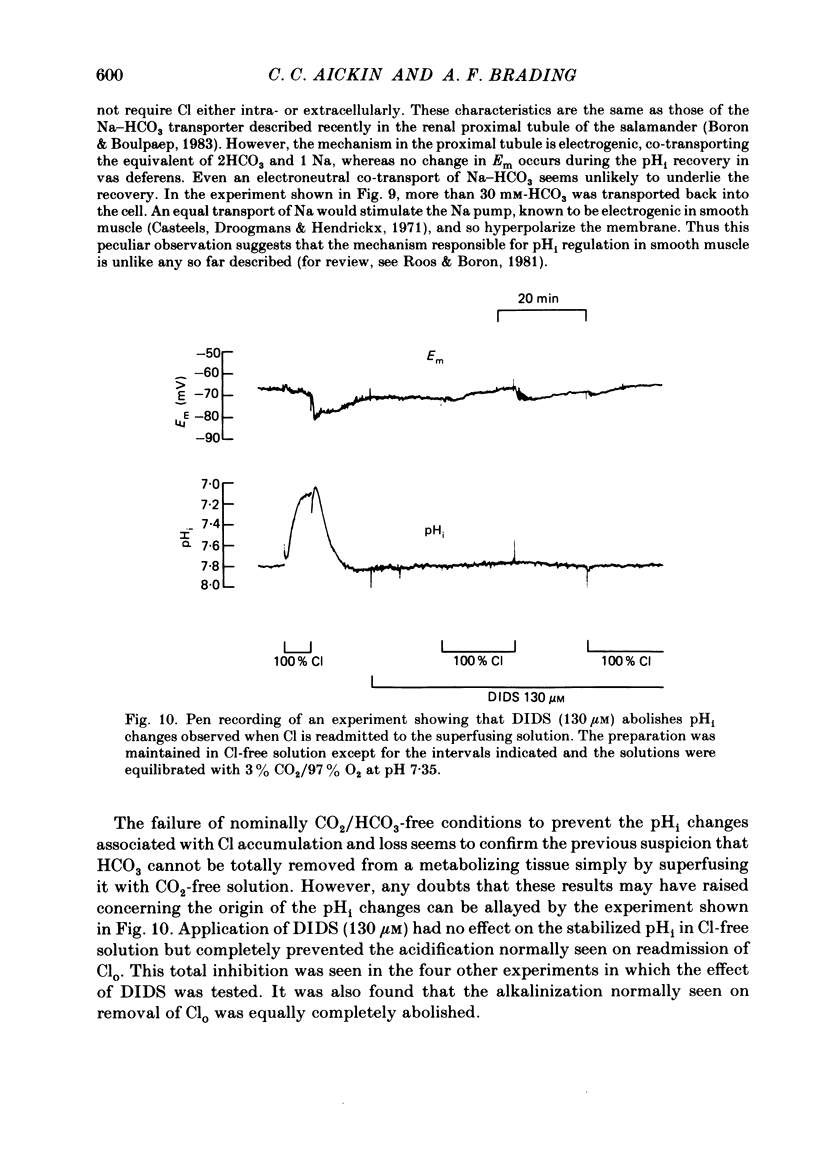
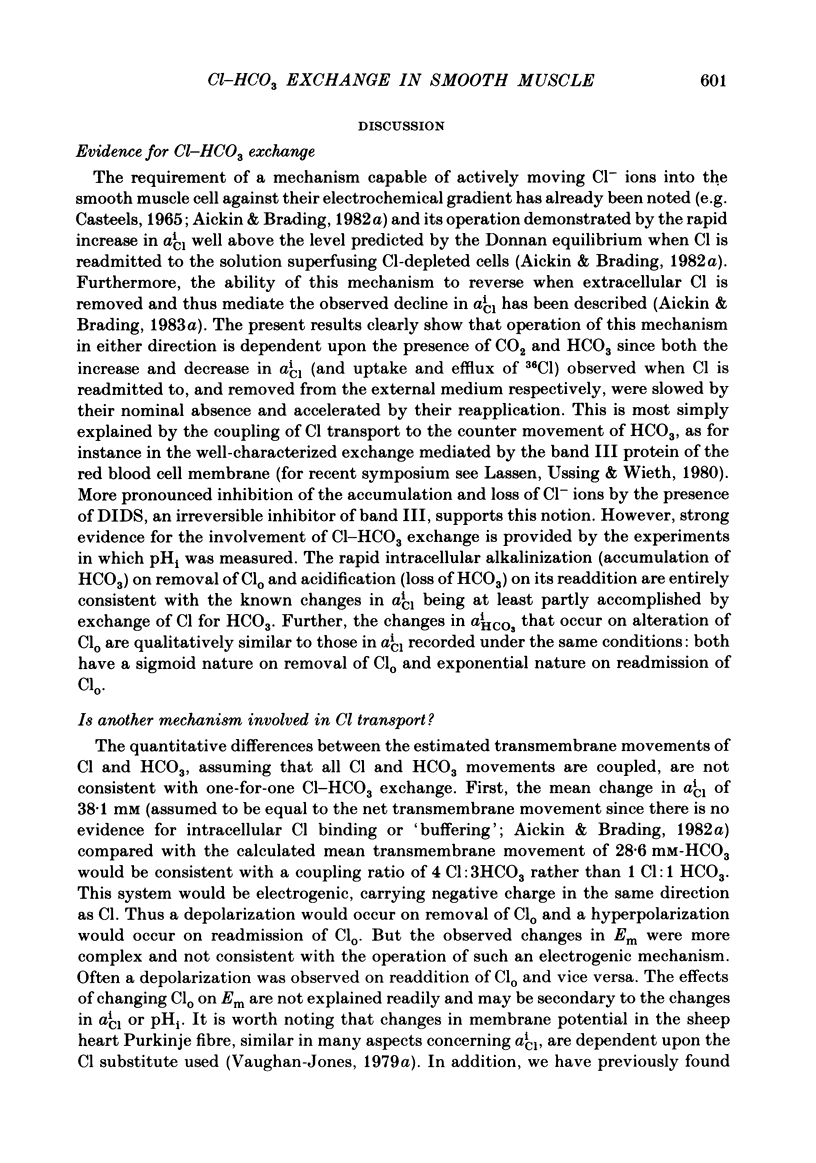
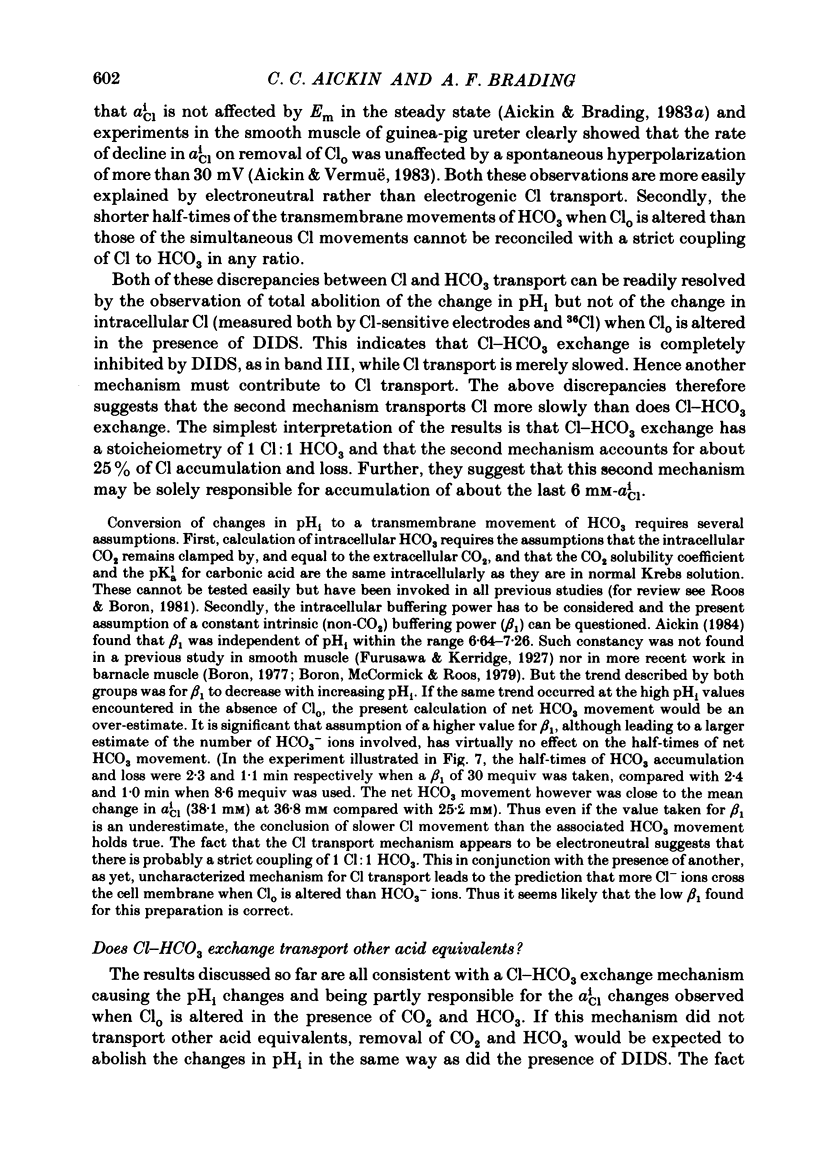
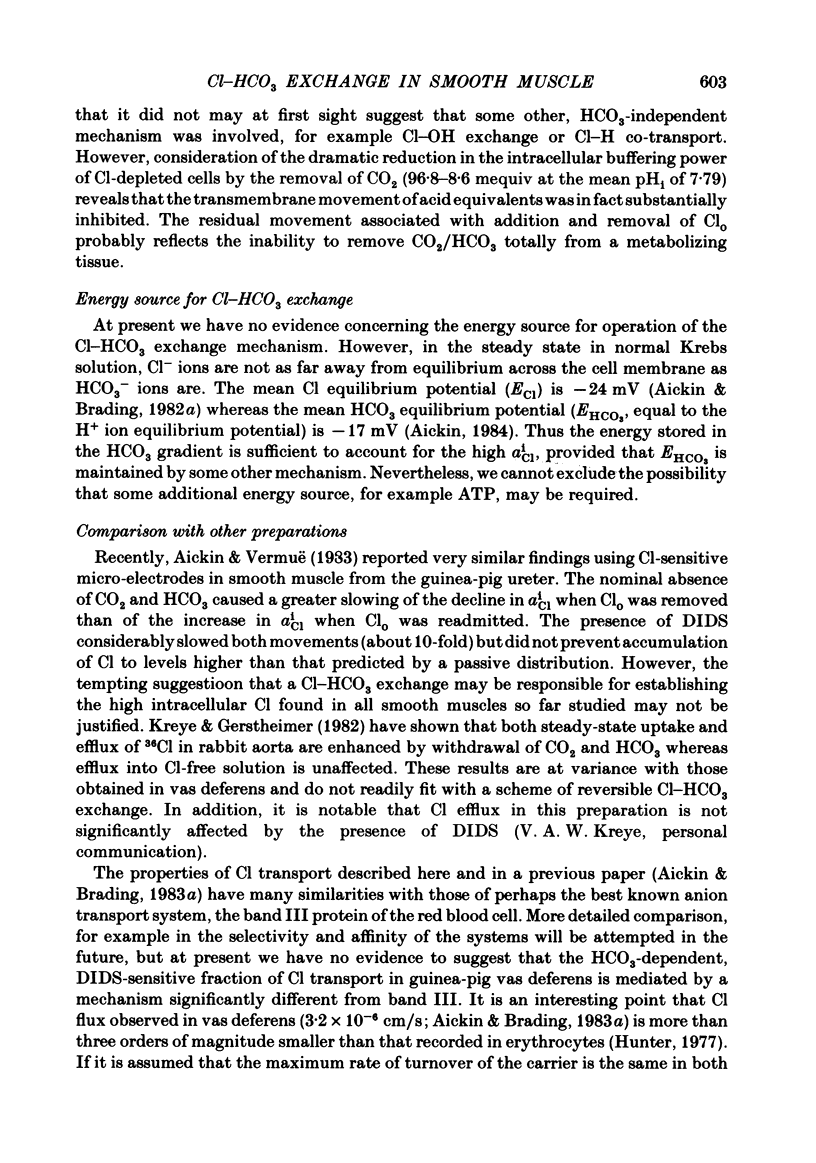
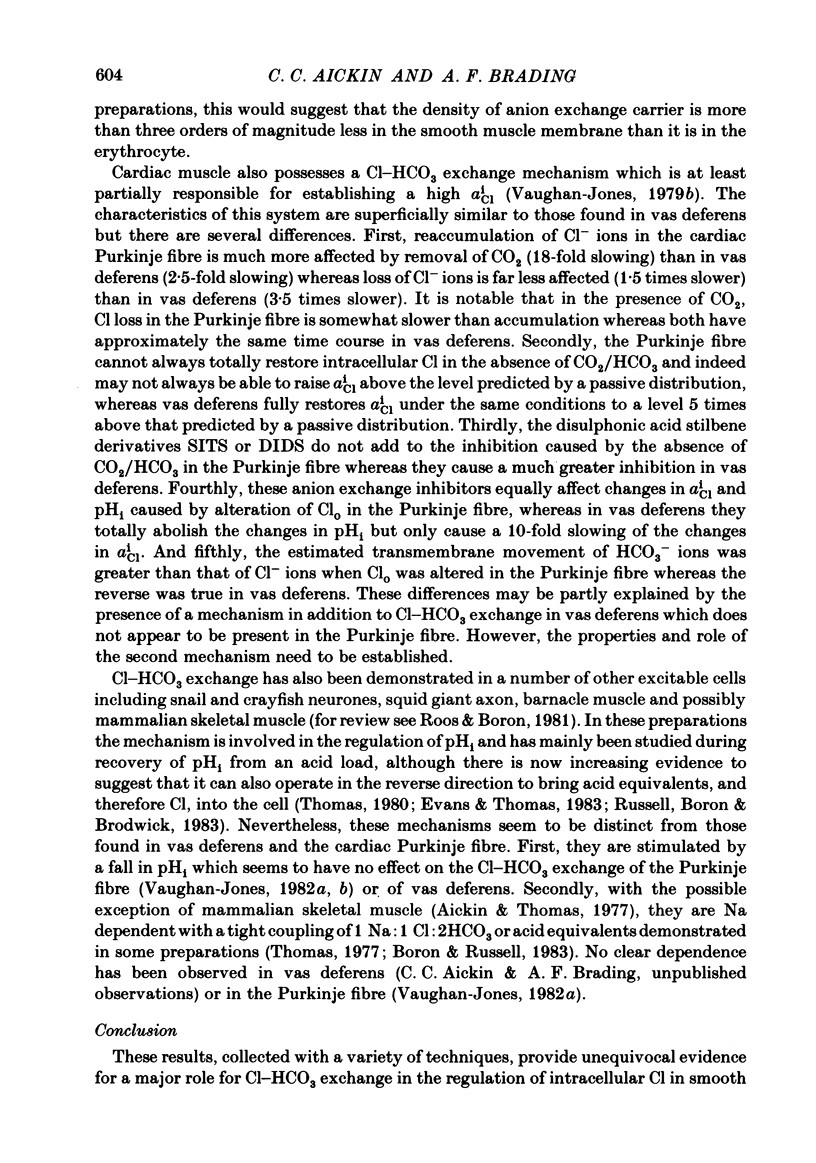
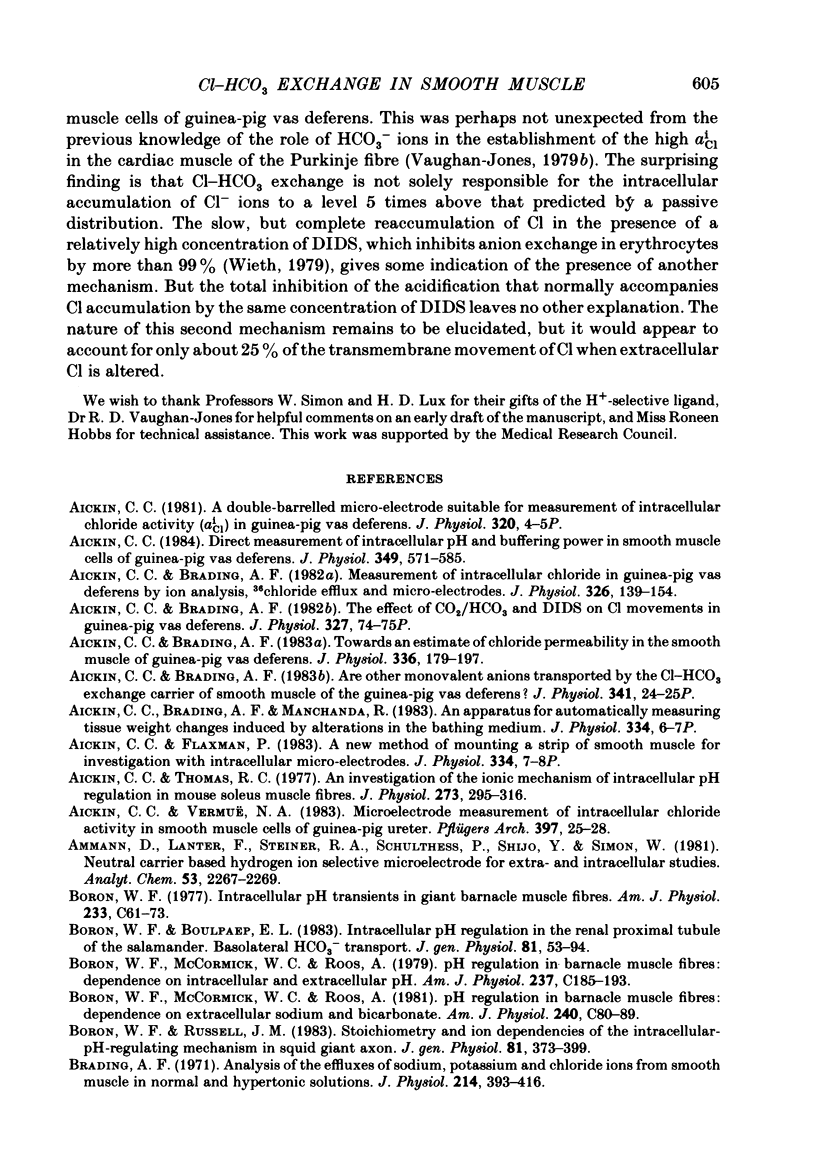
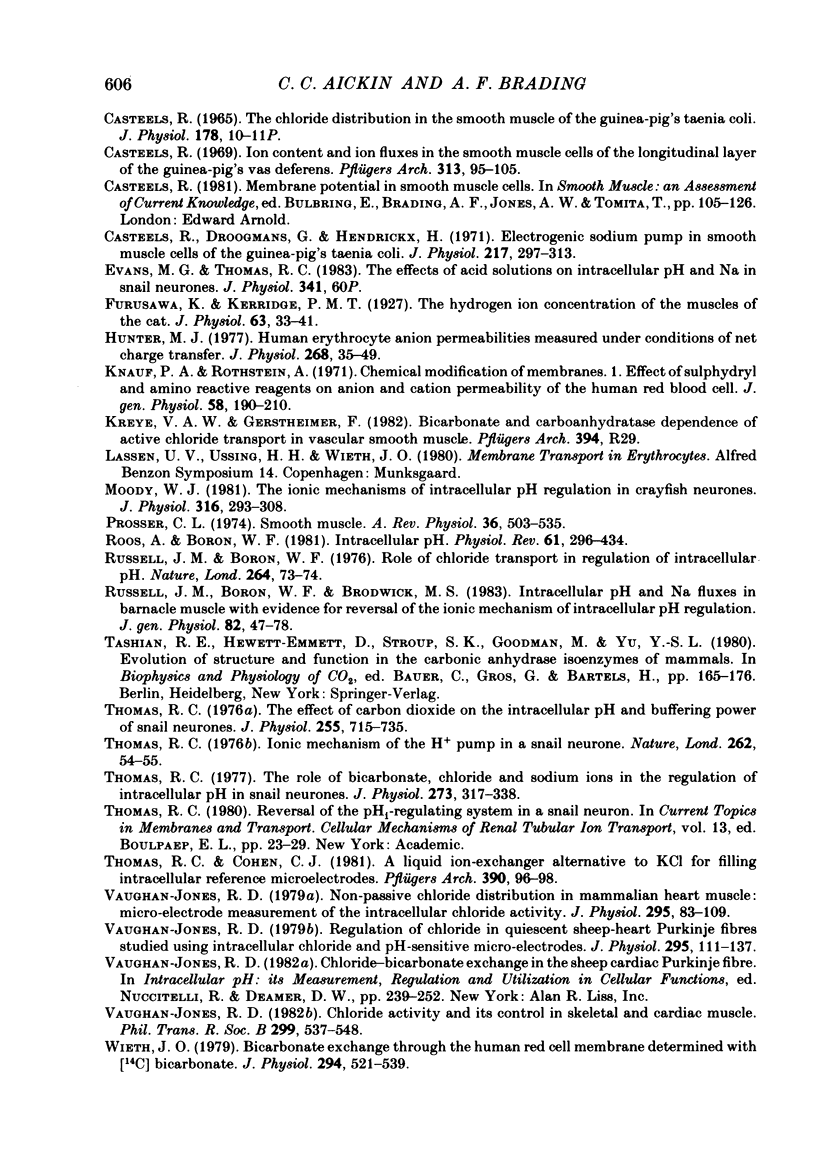
Selected References
These references are in PubMed. This may not be the complete list of references from this article.
- Aickin C. C., Brading A. F. Measurement of intracellular chloride in guinea-pig vas deferens by ion analysis, 36chloride efflux and micro-electrodes. J Physiol. 1982 May;326:139–154. doi: 10.1113/jphysiol.1982.sp014182. [DOI] [PMC free article] [PubMed] [Google Scholar]
- Aickin C. C., Brading A. F. Towards an estimate of chloride permeability in the smooth muscle of guinea-pig vas deferens. J Physiol. 1983 Mar;336:179–197. doi: 10.1113/jphysiol.1983.sp014575. [DOI] [PMC free article] [PubMed] [Google Scholar]
- Aickin C. C. Direct measurement of intracellular pH and buffering power in smooth muscle cells of guinea-pig vas deferens. J Physiol. 1984 Apr;349:571–585. doi: 10.1113/jphysiol.1984.sp015174. [DOI] [PMC free article] [PubMed] [Google Scholar]
- Aickin C. C., Thomas R. C. An investigation of the ionic mechanism of intracellular pH regulation in mouse soleus muscle fibres. J Physiol. 1977 Dec;273(1):295–316. doi: 10.1113/jphysiol.1977.sp012095. [DOI] [PMC free article] [PubMed] [Google Scholar]
- Aickin C. C., Vermuë N. A. Microelectrode measurement of intracellular chloride activity in smooth muscle cells of guinea-pig ureter. Pflugers Arch. 1983 Apr;397(1):25–28. doi: 10.1007/BF00585163. [DOI] [PubMed] [Google Scholar]
- Ammann D., Lanter F., Steiner R. A., Schulthess P., Shijo Y., Simon W. Neutral carrier based hydrogen ion selective microelectrode for extra- and intracellular studies. Anal Chem. 1981 Dec;53(14):2267–2269. doi: 10.1021/ac00237a031. [DOI] [PubMed] [Google Scholar]
- Boron W. F., Boulpaep E. L. Intracellular pH regulation in the renal proximal tubule of the salamander. Basolateral HCO3- transport. J Gen Physiol. 1983 Jan;81(1):53–94. doi: 10.1085/jgp.81.1.53. [DOI] [PMC free article] [PubMed] [Google Scholar]
- Boron W. F. Intracellular pH transients in giant barnacle muscle fibers. Am J Physiol. 1977 Sep;233(3):C61–C73. doi: 10.1152/ajpcell.1977.233.3.C61. [DOI] [PubMed] [Google Scholar]
- Boron W. F., McCormick W. C., Roos A. pH regulation in barnacle muscle fibers: dependence on intracellular and extracellular pH. Am J Physiol. 1979 Sep;237(3):C185–C193. doi: 10.1152/ajpcell.1979.237.3.C185. [DOI] [PubMed] [Google Scholar]
- Boron W. F., Russell J. M. Stoichiometry and ion dependencies of the intracellular-pH-regulating mechanism in squid giant axons. J Gen Physiol. 1983 Mar;81(3):373–399. doi: 10.1085/jgp.81.3.373. [DOI] [PMC free article] [PubMed] [Google Scholar]
- Brading A. F. Analysis of the effluxes of sodium, potassium and chloride ions from smooth muscle in normal and hypertonic solutions. J Physiol. 1971 May;214(3):393–416. doi: 10.1113/jphysiol.1971.sp009440. [DOI] [PMC free article] [PubMed] [Google Scholar]
- Casteels R., Droogmans G., Hendrickx H. Electrogenic sodium pump in smooth muscle cells of the guinea-pig's taenia coli. J Physiol. 1971 Sep;217(2):297–313. doi: 10.1113/jphysiol.1971.sp009572. [DOI] [PMC free article] [PubMed] [Google Scholar]
- Casteels R. Ion content and ion fluxes in the smooth muscle cells of the longitudinal layer of the guinea-pig's vas deferens. Pflugers Arch. 1969;313(2):95–105. doi: 10.1007/BF00586238. [DOI] [PubMed] [Google Scholar]
- Furusawa K., Kerridge P. M. The hydrogen ion concentration of the muscles of the cat. J Physiol. 1927 Jun 7;63(1):33–41. doi: 10.1113/jphysiol.1927.sp002378. [DOI] [PMC free article] [PubMed] [Google Scholar]
- Hunter M. J. Human erythrocyte anion permeabilities measured under conditions of net charge transfer. J Physiol. 1977 Jun;268(1):35–49. doi: 10.1113/jphysiol.1977.sp011845. [DOI] [PMC free article] [PubMed] [Google Scholar]
- Knauf P. A., Rothstein A. Chemical modification of membranes. I. Effects of sulfhydryl and amino reactive reagents on anion and cation permeability of the human red blood cell. J Gen Physiol. 1971 Aug;58(2):190–210. doi: 10.1085/jgp.58.2.190. [DOI] [PMC free article] [PubMed] [Google Scholar]
- Moody W. J., Jr The ionic mechanism of intracellular pH regulation in crayfish neurones. J Physiol. 1981 Jul;316:293–308. doi: 10.1113/jphysiol.1981.sp013788. [DOI] [PMC free article] [PubMed] [Google Scholar]
- Prosser C. L. Smooth muscle. Annu Rev Physiol. 1974;36:503–535. doi: 10.1146/annurev.ph.36.030174.002443. [DOI] [PubMed] [Google Scholar]
- Roos A., Boron W. F. Intracellular pH. Physiol Rev. 1981 Apr;61(2):296–434. doi: 10.1152/physrev.1981.61.2.296. [DOI] [PubMed] [Google Scholar]
- Russell J. M., Boron W. F., Brodwick M. S. Intracellular pH and Na fluxes in barnacle muscle with evidence for reversal of the ionic mechanism of intracellular pH regulation. J Gen Physiol. 1983 Jul;82(1):47–78. doi: 10.1085/jgp.82.1.47. [DOI] [PMC free article] [PubMed] [Google Scholar]
- Russell J. M., Boron W. F. Role of choloride transport in regulation of intracellular pH. Nature. 1976 Nov 4;264(5581):73–74. doi: 10.1038/264073a0. [DOI] [PubMed] [Google Scholar]
- Thomas R. C., Cohen C. J. A liquid ion-exchanger alternative to KCl for filling intracellular reference microelectrodes. Pflugers Arch. 1981 Apr;390(1):96–98. doi: 10.1007/BF00582719. [DOI] [PubMed] [Google Scholar]
- Thomas R. C. Ionic mechanism of the H+ pump in a snail neurone. Nature. 1976 Jul 1;262(5563):54–55. doi: 10.1038/262054a0. [DOI] [PubMed] [Google Scholar]
- Thomas R. C. The effect of carbon dioxide on the intracellular pH and buffering power of snail neurones. J Physiol. 1976 Mar;255(3):715–735. doi: 10.1113/jphysiol.1976.sp011305. [DOI] [PMC free article] [PubMed] [Google Scholar]
- Thomas R. C. The role of bicarbonate, chloride and sodium ions in the regulation of intracellular pH in snail neurones. J Physiol. 1977 Dec;273(1):317–338. doi: 10.1113/jphysiol.1977.sp012096. [DOI] [PMC free article] [PubMed] [Google Scholar]
- Vaughan-Jones R. D. Chloride activity and its control in skeletal and cardiac muscle. Philos Trans R Soc Lond B Biol Sci. 1982 Dec 1;299(1097):537–548. doi: 10.1098/rstb.1982.0150. [DOI] [PubMed] [Google Scholar]
- Vaughan-Jones R. D. Non-passive chloride distribution in mammalian heart muscle: micro-electrode measurement of the intracellular chloride activity. J Physiol. 1979 Oct;295:83–109. doi: 10.1113/jphysiol.1979.sp012956. [DOI] [PMC free article] [PubMed] [Google Scholar]
- Vaughan-Jones R. D. Regulation of chloride in quiescent sheep-heart Purkinje fibres studied using intracellular chloride and pH-sensitive micro-electrodes. J Physiol. 1979 Oct;295:111–137. doi: 10.1113/jphysiol.1979.sp012957. [DOI] [PMC free article] [PubMed] [Google Scholar]
- Wieth J. O. Bicarbonate exchange through the human red cell membrane determined with [14C] bicarbonate. J Physiol. 1979 Sep;294:521–539. doi: 10.1113/jphysiol.1979.sp012944. [DOI] [PMC free article] [PubMed] [Google Scholar]