Abstract
A modified Poisson-Boltzmann equation is developed from statistical mechanical considerations to describe the influence of the transmembrane potential on macromolecular systems. Using a Green's function formalism, the electrostatic free energy of a protein associated with the membrane is expressed as the sum of three terms: a contribution from the energy required to charge the system's capacitance, a contribution corresponding to the interaction of the protein charges with the membrane potential, and a contribution corresponding to a voltage-independent reaction field free energy. The membrane potential, which is due to the polarization interface, is calculated in the absence of the protein charges, whereas the reaction field is calculated in the absence of transmembrane potential. Variations in the capacitive energy associated with typical molecular processes are negligible under physiological conditions. The formulation of the theory is closely related to standard algorithms used to solve the Poisson-Boltzmann equation and only small modifications to current source codes are required for its implementation. The theory is illustrated by examining the voltage-dependent membrane insertion of a simple polyalanine alpha-helix and by computing the electrostatic potential across a 60-A-diameter sphere meant to represent a large intrinsic protein.
Full text
PDF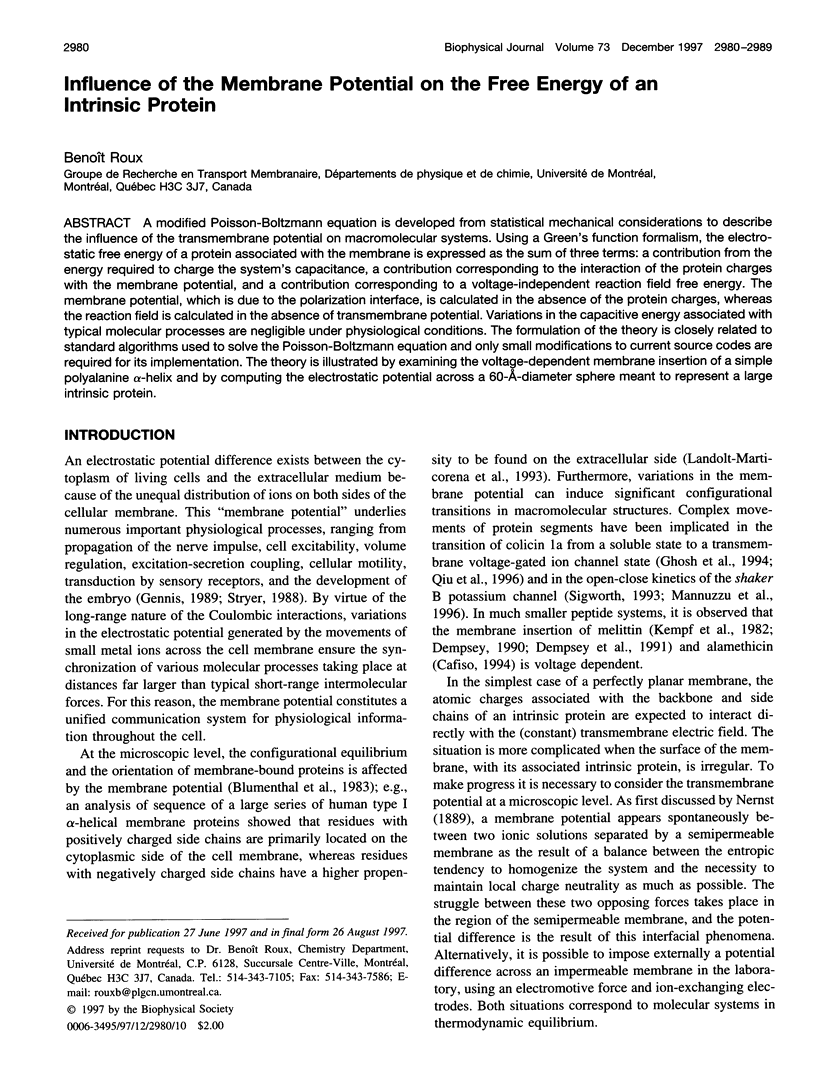
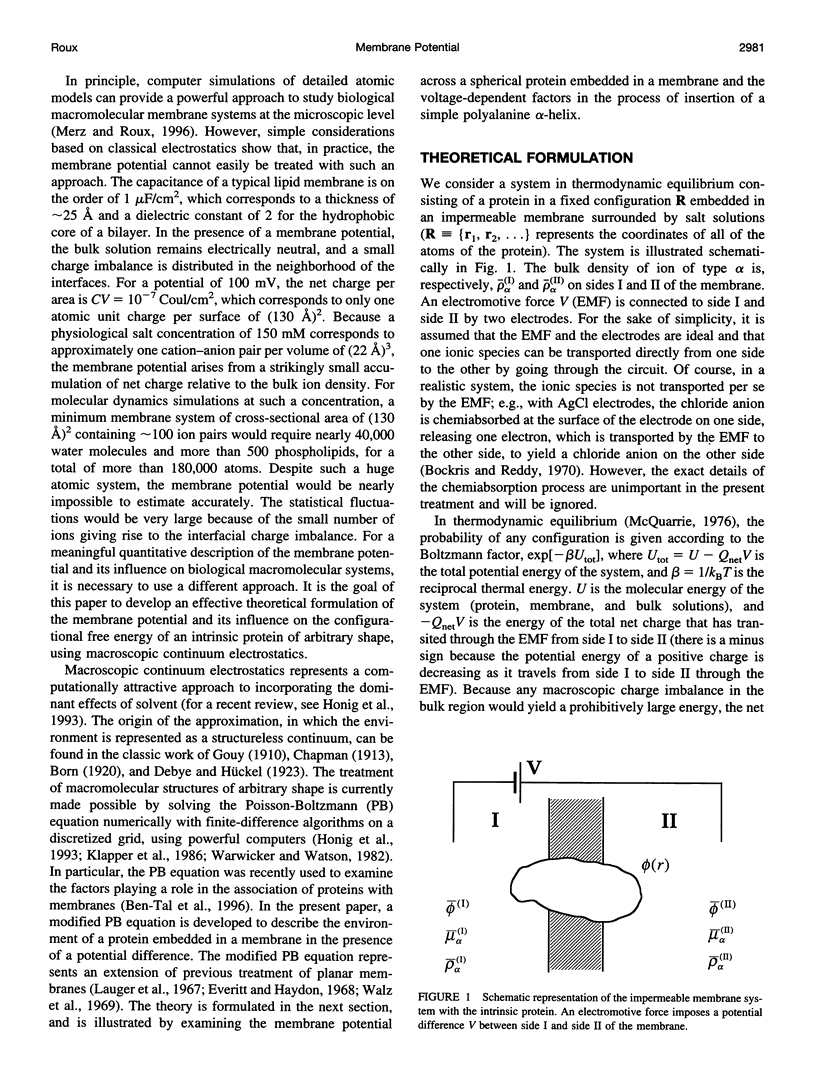
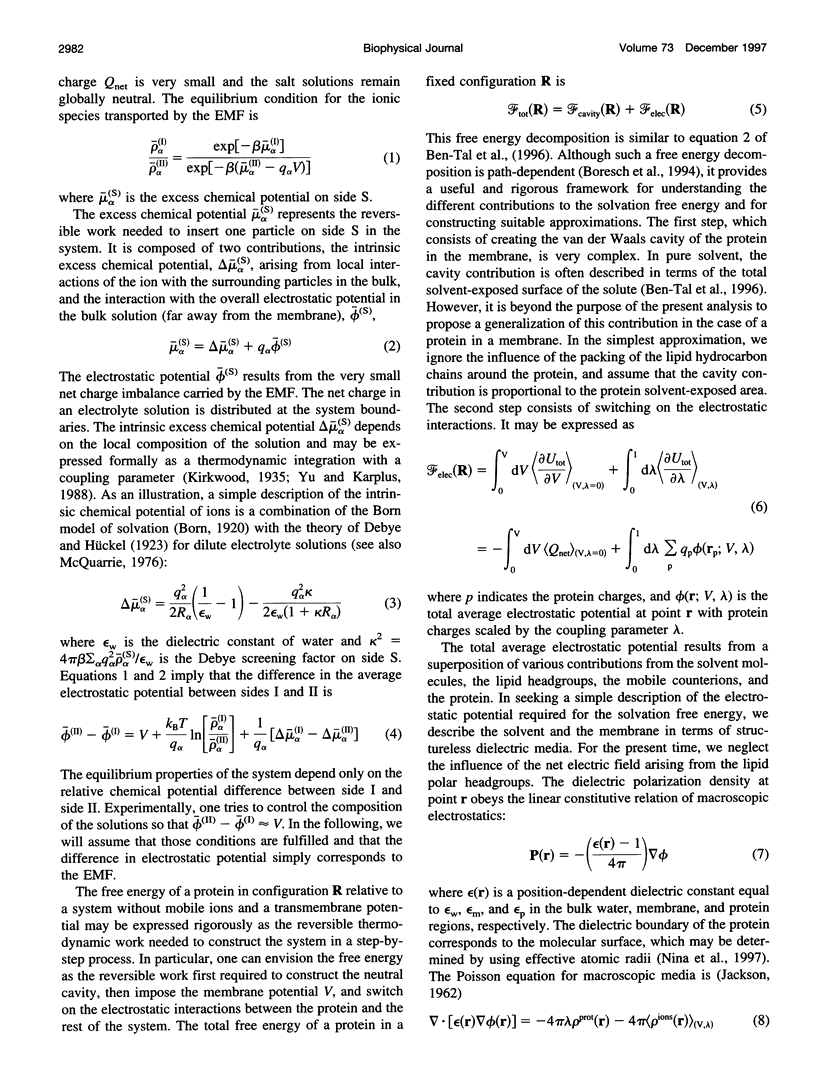
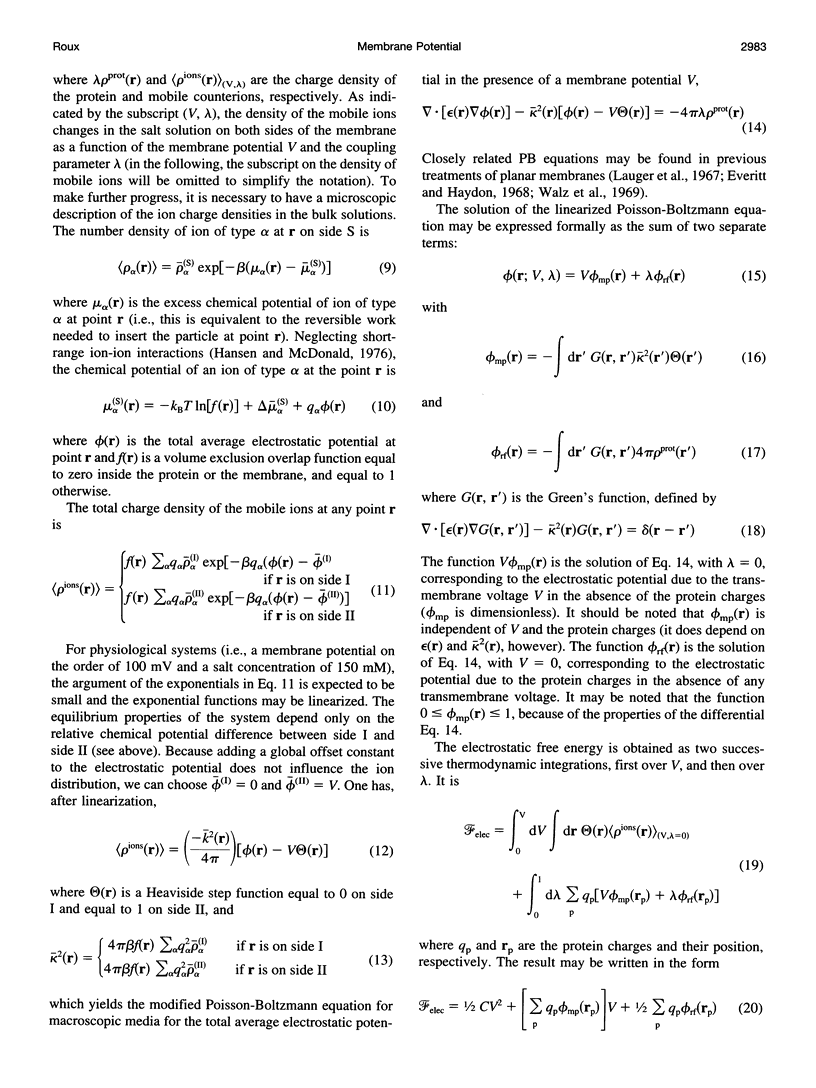
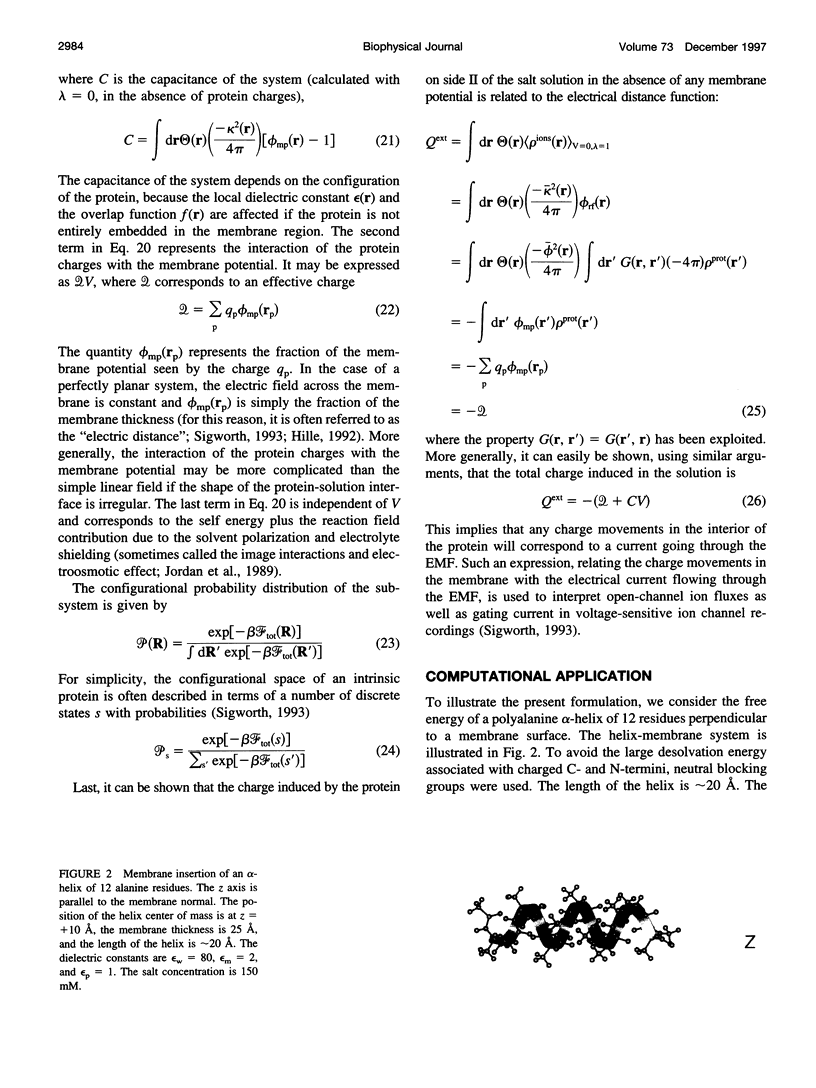
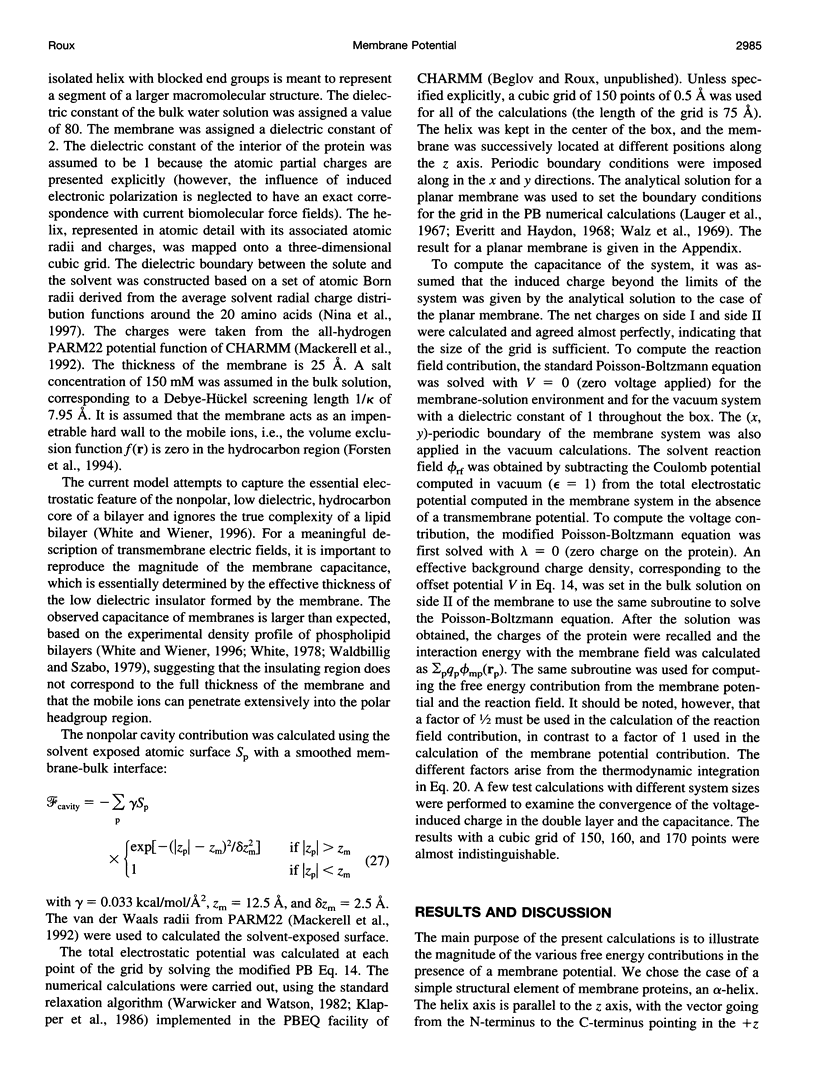
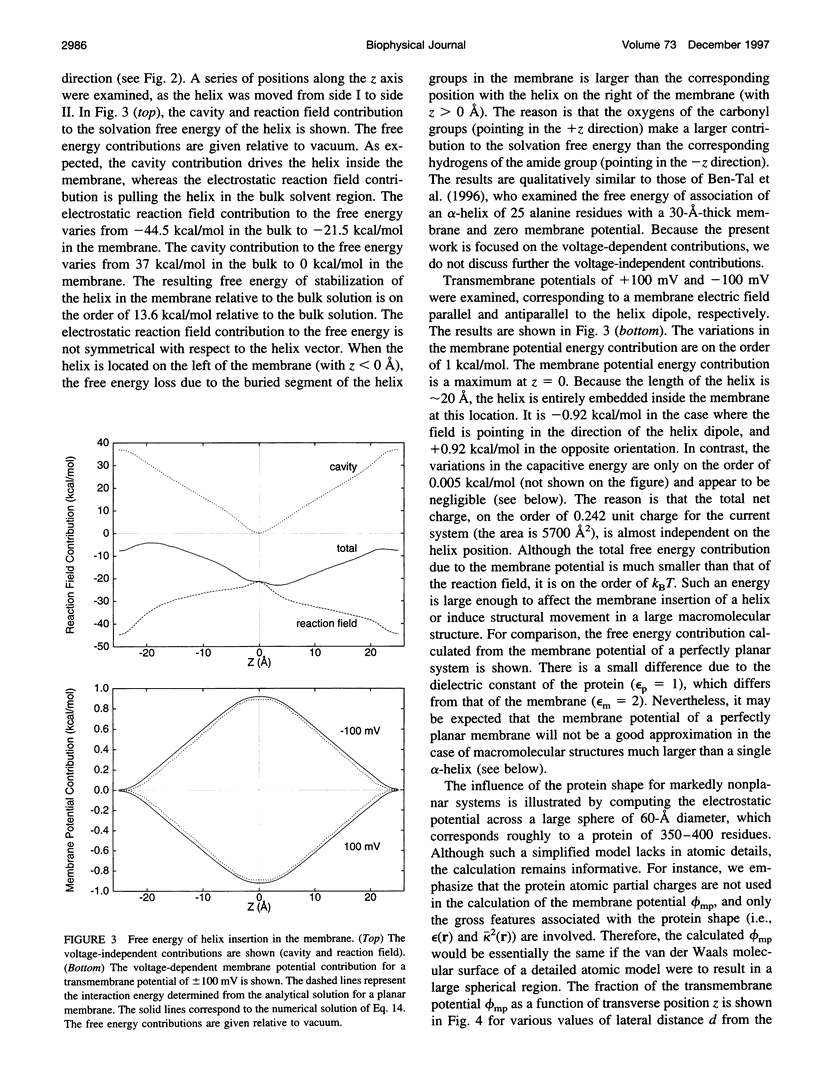
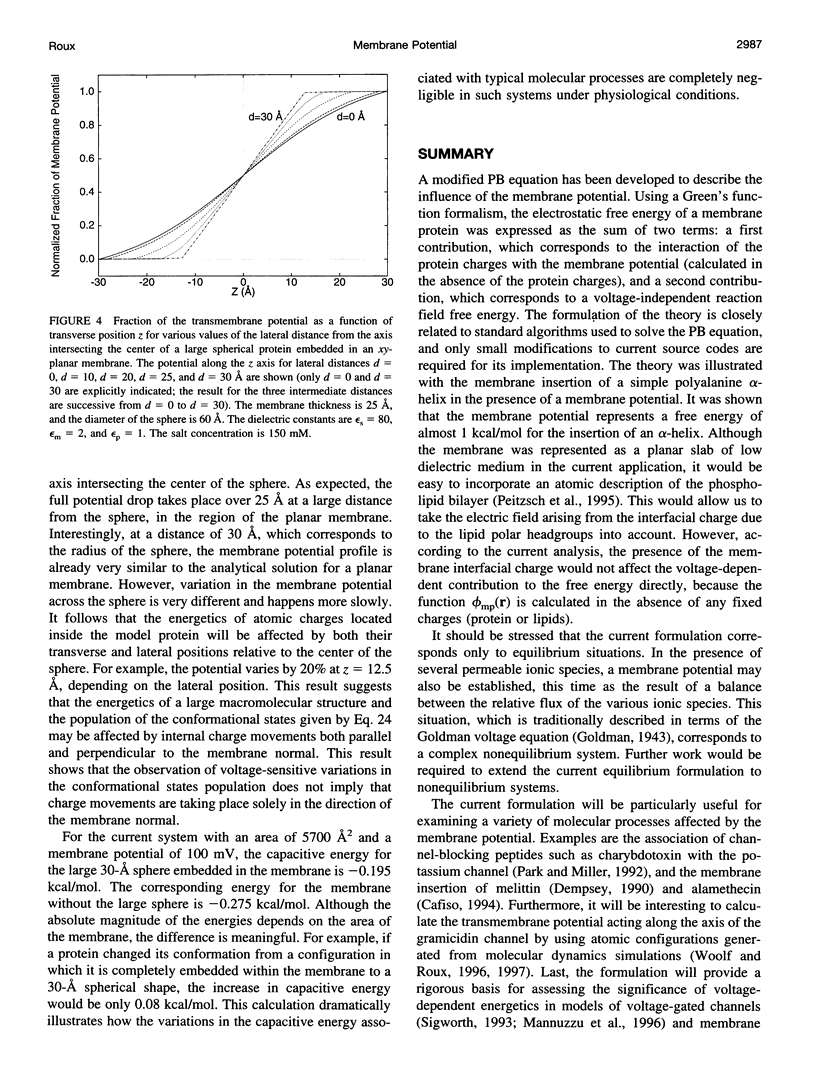
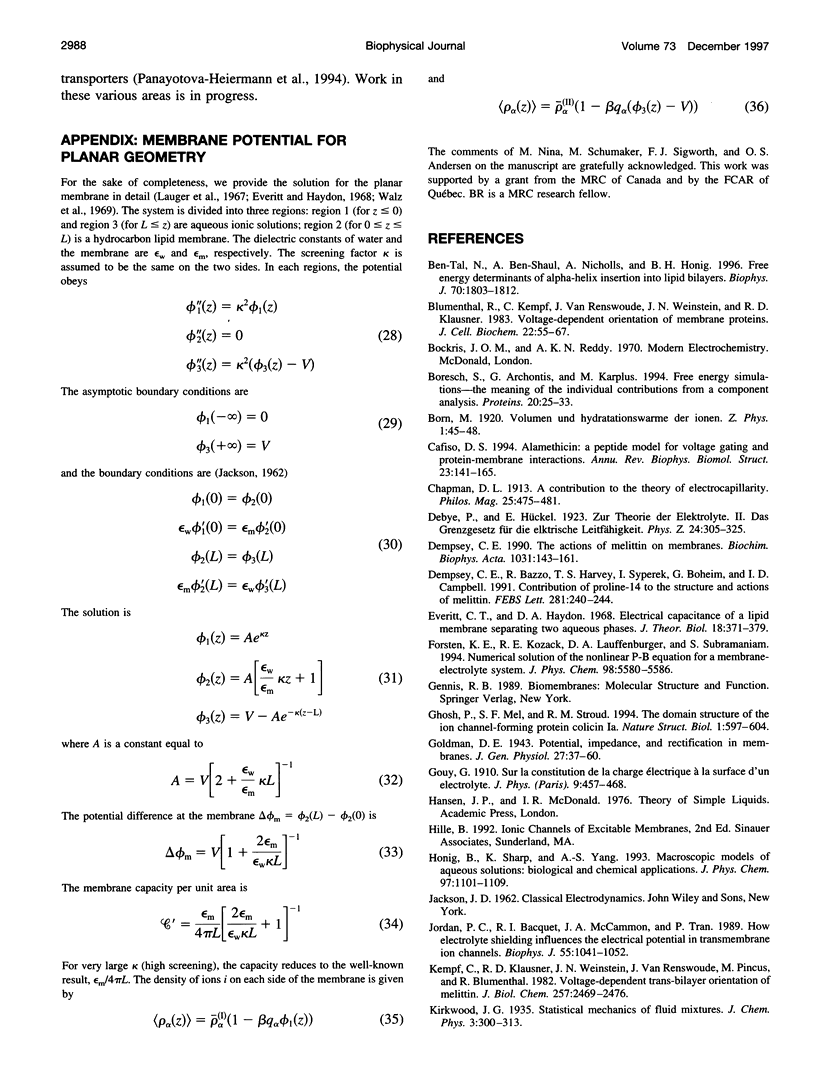
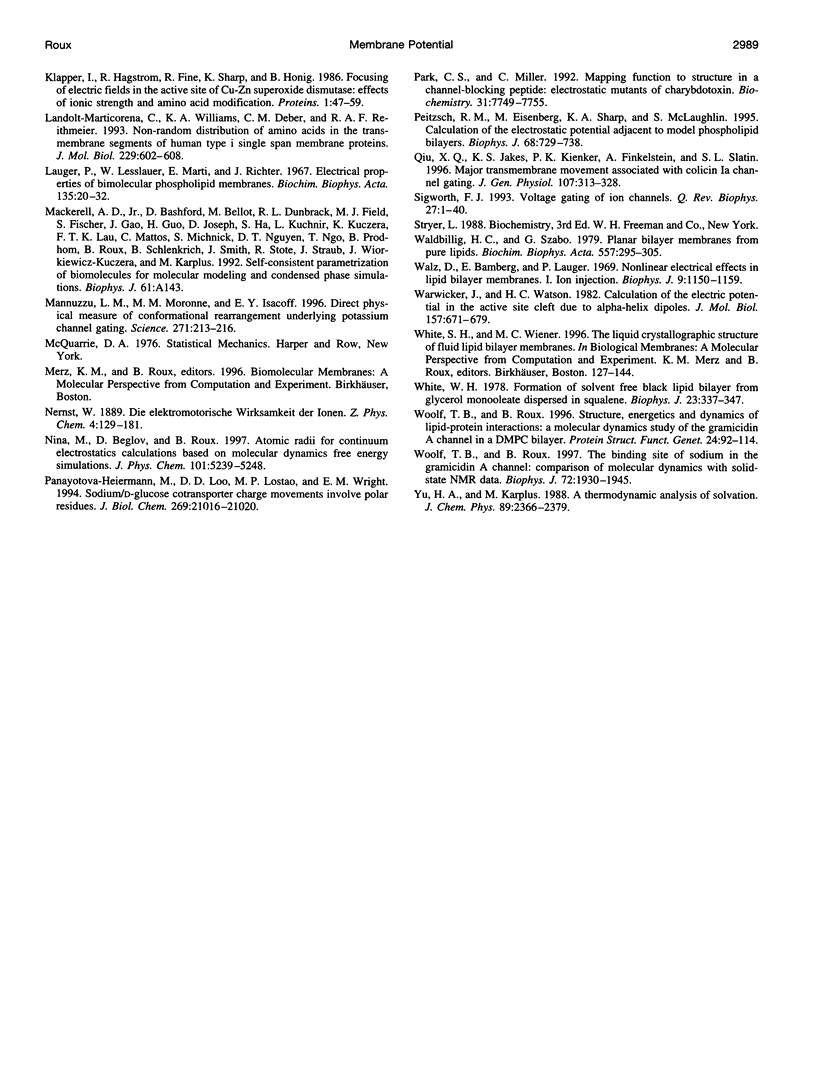
Images in this article
Selected References
These references are in PubMed. This may not be the complete list of references from this article.
- Ben-Tal N., Ben-Shaul A., Nicholls A., Honig B. Free-energy determinants of alpha-helix insertion into lipid bilayers. Biophys J. 1996 Apr;70(4):1803–1812. doi: 10.1016/S0006-3495(96)79744-8. [DOI] [PMC free article] [PubMed] [Google Scholar]
- Blumenthal R., Kempf C., Van Renswoude J., Weinstein J. N., Klausner R. D. Voltage-dependent orientation of membrane proteins. J Cell Biochem. 1983;22(1):55–67. doi: 10.1002/jcb.240220106. [DOI] [PubMed] [Google Scholar]
- Boresch S., Archontis G., Karplus M. Free energy simulations: the meaning of the individual contributions from a component analysis. Proteins. 1994 Sep;20(1):25–33. doi: 10.1002/prot.340200105. [DOI] [PubMed] [Google Scholar]
- Cafiso D. S. Alamethicin: a peptide model for voltage gating and protein-membrane interactions. Annu Rev Biophys Biomol Struct. 1994;23:141–165. doi: 10.1146/annurev.bb.23.060194.001041. [DOI] [PubMed] [Google Scholar]
- Dempsey C. E., Bazzo R., Harvey T. S., Syperek I., Boheim G., Campbell I. D. Contribution of proline-14 to the structure and actions of melittin. FEBS Lett. 1991 Apr 9;281(1-2):240–244. doi: 10.1016/0014-5793(91)80402-o. [DOI] [PubMed] [Google Scholar]
- Dempsey C. E. The actions of melittin on membranes. Biochim Biophys Acta. 1990 May 7;1031(2):143–161. doi: 10.1016/0304-4157(90)90006-x. [DOI] [PubMed] [Google Scholar]
- Everitt C. T., Haydon D. A. Electrical capacitance of a lipid membrane separating two aqueous phases. J Theor Biol. 1968 Mar;18(3):371–379. doi: 10.1016/0022-5193(68)90084-2. [DOI] [PubMed] [Google Scholar]
- Ghosh P., Mel S. F., Stroud R. M. The domain structure of the ion channel-forming protein colicin Ia. Nat Struct Biol. 1994 Sep;1(9):597–604. doi: 10.1038/nsb0994-597. [DOI] [PubMed] [Google Scholar]
- Jordan P. C., Bacquet R. J., McCammon J. A., Tran P. How electrolyte shielding influences the electrical potential in transmembrane ion channels. Biophys J. 1989 Jun;55(6):1041–1052. doi: 10.1016/S0006-3495(89)82903-0. [DOI] [PMC free article] [PubMed] [Google Scholar]
- Kempf C., Klausner R. D., Weinstein J. N., Van Renswoude J., Pincus M., Blumenthal R. Voltage-dependent trans-bilayer orientation of melittin. J Biol Chem. 1982 Mar 10;257(5):2469–2476. [PubMed] [Google Scholar]
- Klapper I., Hagstrom R., Fine R., Sharp K., Honig B. Focusing of electric fields in the active site of Cu-Zn superoxide dismutase: effects of ionic strength and amino-acid modification. Proteins. 1986 Sep;1(1):47–59. doi: 10.1002/prot.340010109. [DOI] [PubMed] [Google Scholar]
- Landolt-Marticorena C., Williams K. A., Deber C. M., Reithmeier R. A. Non-random distribution of amino acids in the transmembrane segments of human type I single span membrane proteins. J Mol Biol. 1993 Feb 5;229(3):602–608. doi: 10.1006/jmbi.1993.1066. [DOI] [PubMed] [Google Scholar]
- Läuger P., Lesslauer W., Marti E., Richter J. Electrical properties of bimolecular phospholipid membranes. Biochim Biophys Acta. 1967 Feb 1;135(1):20–32. doi: 10.1016/0005-2736(67)90004-1. [DOI] [PubMed] [Google Scholar]
- Mannuzzu L. M., Moronne M. M., Isacoff E. Y. Direct physical measure of conformational rearrangement underlying potassium channel gating. Science. 1996 Jan 12;271(5246):213–216. doi: 10.1126/science.271.5246.213. [DOI] [PubMed] [Google Scholar]
- Panayotova-Heiermann M., Loo D. D., Lostao M. P., Wright E. M. Sodium/D-glucose cotransporter charge movements involve polar residues. J Biol Chem. 1994 Aug 19;269(33):21016–21020. [PubMed] [Google Scholar]
- Park C. S., Miller C. Mapping function to structure in a channel-blocking peptide: electrostatic mutants of charybdotoxin. Biochemistry. 1992 Sep 1;31(34):7749–7755. doi: 10.1021/bi00149a002. [DOI] [PubMed] [Google Scholar]
- Peitzsch R. M., Eisenberg M., Sharp K. A., McLaughlin S. Calculations of the electrostatic potential adjacent to model phospholipid bilayers. Biophys J. 1995 Mar;68(3):729–738. doi: 10.1016/S0006-3495(95)80253-5. [DOI] [PMC free article] [PubMed] [Google Scholar]
- Qiu X. Q., Jakes K. S., Kienker P. K., Finkelstein A., Slatin S. L. Major transmembrane movement associated with colicin Ia channel gating. J Gen Physiol. 1996 Mar;107(3):313–328. doi: 10.1085/jgp.107.3.313. [DOI] [PMC free article] [PubMed] [Google Scholar]
- Sigworth F. J. Voltage gating of ion channels. Q Rev Biophys. 1994 Feb;27(1):1–40. doi: 10.1017/s0033583500002894. [DOI] [PubMed] [Google Scholar]
- Waldbillig R. C., Szabo G. Planar bilayer membranes from pure lipids. Biochim Biophys Acta. 1979 Nov 2;557(2):295–305. doi: 10.1016/0005-2736(79)90328-6. [DOI] [PubMed] [Google Scholar]
- Walz D., Bamberg E., Läuger P. Nonlinear electrical effects in lipid bilayer membranes. I. Ion injection. Biophys J. 1969 Sep;9(9):1150–1159. doi: 10.1016/S0006-3495(69)86442-8. [DOI] [PMC free article] [PubMed] [Google Scholar]
- Warwicker J., Watson H. C. Calculation of the electric potential in the active site cleft due to alpha-helix dipoles. J Mol Biol. 1982 Jun 5;157(4):671–679. doi: 10.1016/0022-2836(82)90505-8. [DOI] [PubMed] [Google Scholar]
- White S. H. Formation of "solvent-free" black lipid bilayer membranes from glyceryl monooleate dispersed in squalene. Biophys J. 1978 Sep;23(3):337–347. doi: 10.1016/S0006-3495(78)85453-8. [DOI] [PMC free article] [PubMed] [Google Scholar]
- Woolf T. B., Roux B. Structure, energetics, and dynamics of lipid-protein interactions: A molecular dynamics study of the gramicidin A channel in a DMPC bilayer. Proteins. 1996 Jan;24(1):92–114. doi: 10.1002/(SICI)1097-0134(199601)24:1<92::AID-PROT7>3.0.CO;2-Q. [DOI] [PubMed] [Google Scholar]
- Woolf T. B., Roux B. The binding site of sodium in the gramicidin A channel: comparison of molecular dynamics with solid-state NMR data. Biophys J. 1997 May;72(5):1930–1945. doi: 10.1016/S0006-3495(97)78839-8. [DOI] [PMC free article] [PubMed] [Google Scholar]