Dynamic allostery drives autocrine and paracrine TGF-β signaling
- PMID: 39288764
- PMCID: PMC11531391
- DOI: 10.1016/j.cell.2024.08.036
Dynamic allostery drives autocrine and paracrine TGF-β signaling
Abstract
TGF-β, essential for development and immunity, is expressed as a latent complex (L-TGF-β) non-covalently associated with its prodomain and presented on immune cell surfaces by covalent association with GARP. Binding to integrin αvβ8 activates L-TGF-β1/GARP. The dogma is that mature TGF-β must physically dissociate from L-TGF-β1 for signaling to occur. Our previous studies discovered that αvβ8-mediated TGF-β autocrine signaling can occur without TGF-β1 release from its latent form. Here, we show that mice engineered to express TGF-β1 that cannot release from L-TGF-β1 survive without early lethal tissue inflammation, unlike those with TGF-β1 deficiency. Combining cryogenic electron microscopy with cell-based assays, we reveal a dynamic allosteric mechanism of autocrine TGF-β1 signaling without release where αvβ8 binding redistributes the intrinsic flexibility of L-TGF-β1 to expose TGF-β1 to its receptors. Dynamic allostery explains the TGF-β3 latency/activation mechanism and why TGF-β3 functions distinctly from TGF-β1, suggesting that it broadly applies to other flexible cell surface receptor/ligand systems.
Keywords: TGF-b signaling; TGF-b1; TGF-b3; activation; autocrine signaling; avb8 integrin; dynamic allostery; entropy redistribution; furin; latency; paracrine signaling; regulatory T cells; single-particle cryo-EM.
Copyright © 2024 The Author(s). Published by Elsevier Inc. All rights reserved.
Conflict of interest statement
Declaration of interests S.L.N. is on the scientific advisory board (SAB) of Corbus Pharmaceuticals, LLC. Y.C. is on the SABs of ShuiMu BioSciences Ltd. and Pamplona Therapeutics. A provisional patent has been filed by the Regents of the University of California.
Figures
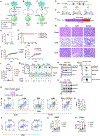
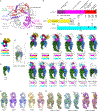
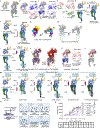
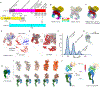
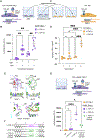
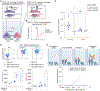
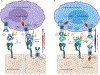
Similar articles
-
Cryo-EM Reveals Integrin-Mediated TGF-β Activation without Release from Latent TGF-β.Cell. 2020 Feb 6;180(3):490-501.e16. doi: 10.1016/j.cell.2019.12.030. Epub 2020 Jan 16. Cell. 2020. PMID: 31955848 Free PMC article.
-
Specificity of TGF-β1 signal designated by LRRC33 and integrin αVβ8.Nat Commun. 2022 Aug 25;13(1):4988. doi: 10.1038/s41467-022-32655-9. Nat Commun. 2022. PMID: 36008481 Free PMC article.
-
Role of GARP in the activation of latent TGF-β1.Mol Biosyst. 2017 Sep 26;13(10):1925-1935. doi: 10.1039/c7mb00251c. Mol Biosyst. 2017. PMID: 28795730 Review.
-
Release of active TGF-β1 from the latent TGF-β1/GARP complex on T regulatory cells is mediated by integrin β8.J Immunol. 2014 Sep 15;193(6):2843-9. doi: 10.4049/jimmunol.1401102. Epub 2014 Aug 15. J Immunol. 2014. PMID: 25127859 Free PMC article.
-
Garp as a therapeutic target for modulation of T regulatory cell function.Expert Opin Ther Targets. 2017 Feb;21(2):191-200. doi: 10.1080/14728222.2017.1275568. Epub 2016 Dec 29. Expert Opin Ther Targets. 2017. PMID: 28001437 Review.
References
-
- Annes JP, Munger JS, and Rifkin DB (2003). Making sense of latent TGFbeta activation. J Cell Sci 116, 217–224. - PubMed
MeSH terms
Substances
Grants and funding
LinkOut - more resources
Full Text Sources