Monocyte-derived macrophages orchestrate multiple cell-type interactions to repair necrotic liver lesions in disease models
- PMID: 37338984
- PMCID: PMC10378165
- DOI: 10.1172/JCI166954
Monocyte-derived macrophages orchestrate multiple cell-type interactions to repair necrotic liver lesions in disease models
Abstract
The liver can fully regenerate after partial resection, and its underlying mechanisms have been extensively studied. The liver can also rapidly regenerate after injury, with most studies focusing on hepatocyte proliferation; however, how hepatic necrotic lesions during acute or chronic liver diseases are eliminated and repaired remains obscure. Here, we demonstrate that monocyte-derived macrophages (MoMFs) were rapidly recruited to and encapsulated necrotic areas during immune-mediated liver injury and that this feature was essential in repairing necrotic lesions. At the early stage of injury, infiltrating MoMFs activated the Jagged1/notch homolog protein 2 (JAG1/NOTCH2) axis to induce cell death-resistant SRY-box transcription factor 9+ (SOX9+) hepatocytes near the necrotic lesions, which acted as a barrier from further injury. Subsequently, necrotic environment (hypoxia and dead cells) induced a cluster of complement 1q-positive (C1q+) MoMFs that promoted necrotic removal and liver repair, while Pdgfb+ MoMFs activated hepatic stellate cells (HSCs) to express α-smooth muscle actin and induce a strong contraction signal (YAP, pMLC) to squeeze and finally eliminate the necrotic lesions. In conclusion, MoMFs play a key role in repairing the necrotic lesions, not only by removing necrotic tissues, but also by inducing cell death-resistant hepatocytes to form a perinecrotic capsule and by activating α-smooth muscle actin-expressing HSCs to facilitate necrotic lesion resolution.
Keywords: Gastroenterology; Hepatitis; Hepatology; Macrophages.
Figures
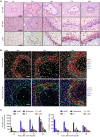
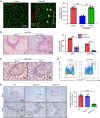
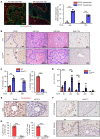
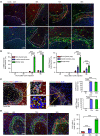
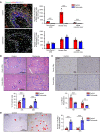
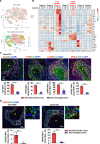
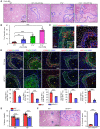
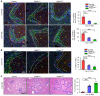
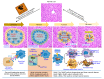
Similar articles
-
Macrophages in necrotic liver lesion repair: opportunities for therapeutical applications.Am J Physiol Cell Physiol. 2024 May 1;326(5):C1556-C1562. doi: 10.1152/ajpcell.00053.2024. Epub 2024 Apr 15. Am J Physiol Cell Physiol. 2024. PMID: 38618702 Review.
-
Hepatic stellate cells orchestrate clearance of necrotic cells in a hypoxia-inducible factor-1α-dependent manner by modulating macrophage phenotype in mice.J Immunol. 2014 Apr 15;192(8):3847-3857. doi: 10.4049/jimmunol.1303195. Epub 2014 Mar 17. J Immunol. 2014. PMID: 24639359 Free PMC article.
-
Distinct spatial distribution and roles of Kupffer cells and monocyte-derived macrophages in mouse acute liver injury.Front Immunol. 2022 Sep 30;13:994480. doi: 10.3389/fimmu.2022.994480. eCollection 2022. Front Immunol. 2022. PMID: 36248843 Free PMC article.
-
Cell-specific PPARγ deficiency establishes anti-inflammatory and anti-fibrogenic properties for this nuclear receptor in non-parenchymal liver cells.J Hepatol. 2013 Nov;59(5):1045-53. doi: 10.1016/j.jhep.2013.06.023. Epub 2013 Jul 2. J Hepatol. 2013. PMID: 23831119
-
Cooperation of liver cells in health and disease.Adv Anat Embryol Cell Biol. 2001;161:III-XIII, 1-151. doi: 10.1007/978-3-642-56553-3. Adv Anat Embryol Cell Biol. 2001. PMID: 11729749 Review.
Cited by
-
The Discovery of Gut Microbial Metabolites as Modulators of Host Susceptibility to Acetaminophen-Induced Hepatotoxicity.Drug Metab Dispos. 2024 Jul 16;52(8):754-764. doi: 10.1124/dmd.123.001541. Drug Metab Dispos. 2024. PMID: 38302428 Review.
-
Macrophages in necrotic liver lesion repair: opportunities for therapeutical applications.Am J Physiol Cell Physiol. 2024 May 1;326(5):C1556-C1562. doi: 10.1152/ajpcell.00053.2024. Epub 2024 Apr 15. Am J Physiol Cell Physiol. 2024. PMID: 38618702 Review.
-
Natural antibodies are required for clearance of necrotic cells and recovery from acute liver injury.JHEP Rep. 2024 Jan 24;6(4):101013. doi: 10.1016/j.jhepr.2024.101013. eCollection 2024 Apr. JHEP Rep. 2024. PMID: 38481390 Free PMC article.
-
Targeting Macrophage Phenotype for Treating Heart Failure: A New Approach.Drug Des Devel Ther. 2024 Nov 5;18:4927-4942. doi: 10.2147/DDDT.S486816. eCollection 2024. Drug Des Devel Ther. 2024. PMID: 39525046 Free PMC article. Review.
-
Targeting cathepsin C ameliorates murine acetaminophen-induced liver injury.Theranostics. 2024 May 13;14(8):3029-3042. doi: 10.7150/thno.96092. eCollection 2024. Theranostics. 2024. PMID: 38855187 Free PMC article.
References
Publication types
MeSH terms
Substances
Grants and funding
LinkOut - more resources
Full Text Sources
Medical
Molecular Biology Databases
Research Materials
Miscellaneous