PIP2 corrects cerebral blood flow deficits in small vessel disease by rescuing capillary Kir2.1 activity
- PMID: 33875602
- PMCID: PMC8092380
- DOI: 10.1073/pnas.2025998118
PIP2 corrects cerebral blood flow deficits in small vessel disease by rescuing capillary Kir2.1 activity
Abstract
Cerebral small vessel diseases (SVDs) are a central link between stroke and dementia-two comorbidities without specific treatments. Despite the emerging consensus that SVDs are initiated in the endothelium, the early mechanisms remain largely unknown. Deficits in on-demand delivery of blood to active brain regions (functional hyperemia) are early manifestations of the underlying pathogenesis. The capillary endothelial cell strong inward-rectifier K+ channel Kir2.1, which senses neuronal activity and initiates a propagating electrical signal that dilates upstream arterioles, is a cornerstone of functional hyperemia. Here, using a genetic SVD mouse model, we show that impaired functional hyperemia is caused by diminished Kir2.1 channel activity. We link Kir2.1 deactivation to depletion of phosphatidylinositol 4,5-bisphosphate (PIP2), a membrane phospholipid essential for Kir2.1 activity. Systemic injection of soluble PIP2 rapidly restored functional hyperemia in SVD mice, suggesting a possible strategy for rescuing functional hyperemia in brain disorders in which blood flow is disturbed.
Keywords: CADASIL; PIP2; cerebral small vessel diseases; functional hyperemia; potassium channel.
Conflict of interest statement
Competing interest statement: Exogenous PIP2 administration was submitted as patent number 62/823,378 titled “Methods to promote cerebral blood flow in the brain” on 25 March 2019.
Figures
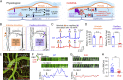
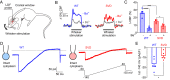
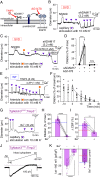
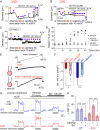
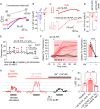
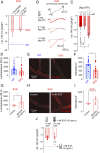
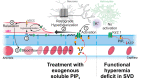
Comment in
-
PIP2 as the "coin of the realm" for neurovascular coupling.Proc Natl Acad Sci U S A. 2021 May 25;118(21):e2106308118. doi: 10.1073/pnas.2106308118. Proc Natl Acad Sci U S A. 2021. PMID: 33952688 Free PMC article. No abstract available.
Similar articles
-
PIP2 Improves Cerebral Blood Flow in a Mouse Model of Alzheimer's Disease.Function (Oxf). 2021 Feb 22;2(2):zqab010. doi: 10.1093/function/zqab010. eCollection 2021. Function (Oxf). 2021. PMID: 33763649 Free PMC article.
-
Endothelial GqPCR activity controls capillary electrical signaling and brain blood flow through PIP2 depletion.Proc Natl Acad Sci U S A. 2018 Apr 10;115(15):E3569-E3577. doi: 10.1073/pnas.1800201115. Epub 2018 Mar 26. Proc Natl Acad Sci U S A. 2018. PMID: 29581272 Free PMC article.
-
Impaired capillary-to-arteriolar electrical signaling after traumatic brain injury.J Cereb Blood Flow Metab. 2021 Jun;41(6):1313-1327. doi: 10.1177/0271678X20962594. Epub 2020 Oct 13. J Cereb Blood Flow Metab. 2021. PMID: 33050826 Free PMC article.
-
Endothelial signaling at the core of neurovascular coupling: The emerging role of endothelial inward-rectifier K+ (Kir2.1) channels and N-methyl-d-aspartate receptors in the regulation of cerebral blood flow.Int J Biochem Cell Biol. 2021 Jun;135:105983. doi: 10.1016/j.biocel.2021.105983. Epub 2021 Apr 21. Int J Biochem Cell Biol. 2021. PMID: 33894355 Review.
-
Activation of inwardly rectifying potassium (Kir) channels by phosphatidylinosital-4,5-bisphosphate (PIP2): interaction with other regulatory ligands.Prog Biophys Mol Biol. 2007 Jul;94(3):320-35. doi: 10.1016/j.pbiomolbio.2006.04.001. Epub 2006 Jun 19. Prog Biophys Mol Biol. 2007. PMID: 16837026 Review.
Cited by
-
Cognition, mood and behavior in CADASIL.Cereb Circ Cogn Behav. 2022 Feb 9;3:100043. doi: 10.1016/j.cccb.2022.100043. eCollection 2022. Cereb Circ Cogn Behav. 2022. PMID: 36324403 Free PMC article.
-
Alterations of the blood-brain barrier during aging.J Cereb Blood Flow Metab. 2024 Jun;44(6):881-895. doi: 10.1177/0271678X241240843. Epub 2024 Mar 21. J Cereb Blood Flow Metab. 2024. PMID: 38513138 Review.
-
Mechanosensory entities and functionality of endothelial cells.Front Cell Dev Biol. 2024 Oct 23;12:1446452. doi: 10.3389/fcell.2024.1446452. eCollection 2024. Front Cell Dev Biol. 2024. PMID: 39507419 Free PMC article. Review.
-
Cerebral Small Vessel Disease-Related Dementia: More Questions Than Answers.Stroke. 2023 Mar;54(3):648-660. doi: 10.1161/STROKEAHA.122.038265. Epub 2023 Feb 27. Stroke. 2023. PMID: 36848423 Free PMC article. Review.
-
Ex vivo ocular perfusion model to study vascular physiology in the mouse eye.Exp Eye Res. 2023 Aug;233:109543. doi: 10.1016/j.exer.2023.109543. Epub 2023 Jun 28. Exp Eye Res. 2023. PMID: 37390954 Free PMC article.
References
-
- Pantoni L., Cerebral small vessel disease: From pathogenesis and clinical characteristics to therapeutic challenges. Lancet Neurol. 9, 689–701 (2010). - PubMed
-
- Wardlaw J. M., Smith C., Dichgans M., Small vessel disease: Mechanisms and clinical implications. Lancet Neurol. 18, 684–696 (2019). - PubMed
-
- Chabriat H., Joutel A., Dichgans M., Tournier-Lasserve E., Bousser M.-G., Cadasil. Lancet Neurol. 8, 643–653 (2009). - PubMed
Publication types
MeSH terms
Substances
Grants and funding
LinkOut - more resources
Full Text Sources
Other Literature Sources
Molecular Biology Databases