Dstyk mutation leads to congenital scoliosis-like vertebral malformations in zebrafish via dysregulated mTORC1/TFEB pathway
- PMID: 31980602
- PMCID: PMC6981171
- DOI: 10.1038/s41467-019-14169-z
Dstyk mutation leads to congenital scoliosis-like vertebral malformations in zebrafish via dysregulated mTORC1/TFEB pathway
Abstract
Congenital scoliosis (CS) is a complex genetic disorder characterized by vertebral malformations. The precise etiology of CS is not fully defined. Here, we identify that mutation in dual serine/threonine and tyrosine protein kinase (dstyk) lead to CS-like vertebral malformations in zebrafish. We demonstrate that the scoliosis in dstyk mutants is related to the wavy and malformed notochord sheath formation and abnormal axial skeleton segmentation due to dysregulated biogenesis of notochord vacuoles and notochord function. Further studies show that DSTYK is located in late endosomal/lysosomal compartments and is involved in the lysosome biogenesis in mammalian cells. Dstyk knockdown inhibits notochord vacuole and lysosome biogenesis through mTORC1-dependent repression of TFEB nuclear translocation. Inhibition of mTORC1 activity can rescue the defect in notochord vacuole biogenesis and scoliosis in dstyk mutants. Together, our findings reveal a key role of DSTYK in notochord vacuole biogenesis, notochord morphogenesis and spine development through mTORC1/TFEB pathway.
Conflict of interest statement
The authors declare no competing interests.
Figures
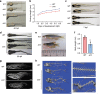
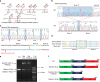
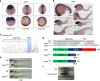
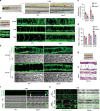
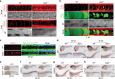
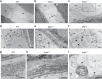
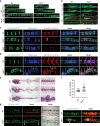
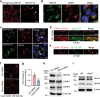
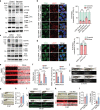
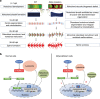
Similar articles
-
Notochord vacuoles absorb compressive bone growth during zebrafish spine formation.Elife. 2020 Jan 29;9:e51221. doi: 10.7554/eLife.51221. Elife. 2020. PMID: 31995030 Free PMC article.
-
ptk7 mutant zebrafish models of congenital and idiopathic scoliosis implicate dysregulated Wnt signalling in disease.Nat Commun. 2014 Sep 3;5:4777. doi: 10.1038/ncomms5777. Nat Commun. 2014. PMID: 25182715 Free PMC article.
-
Notochord vacuoles are lysosome-related organelles that function in axis and spine morphogenesis.J Cell Biol. 2013 Mar 4;200(5):667-79. doi: 10.1083/jcb.201212095. J Cell Biol. 2013. PMID: 23460678 Free PMC article.
-
Disordered vertebral and rib morphology in pudgy mice. Structural relationships to human scoliosis.Adv Anat Embryol Cell Biol. 2016;221:1-123. doi: 10.1007/978-3-319-43151-2_1. Adv Anat Embryol Cell Biol. 2016. PMID: 27655002 Review.
-
On being the right shape: Roles for motile cilia and cerebrospinal fluid flow in body and spine morphology.Semin Cell Dev Biol. 2021 Feb;110:104-112. doi: 10.1016/j.semcdb.2020.07.005. Epub 2020 Jul 18. Semin Cell Dev Biol. 2021. PMID: 32693941 Review.
Cited by
-
MiT/TFE Family of Transcription Factors: An Evolutionary Perspective.Front Cell Dev Biol. 2021 Jan 6;8:609683. doi: 10.3389/fcell.2020.609683. eCollection 2020. Front Cell Dev Biol. 2021. PMID: 33490073 Free PMC article. Review.
-
Zebrafish: an important model for understanding scoliosis.Cell Mol Life Sci. 2022 Sep 4;79(9):506. doi: 10.1007/s00018-022-04534-5. Cell Mol Life Sci. 2022. PMID: 36059018 Free PMC article. Review.
-
Fgfr3 mutation disrupts chondrogenesis and bone ossification in zebrafish model mimicking CATSHL syndrome partially via enhanced Wnt/β-catenin signaling.Theranostics. 2020 May 30;10(16):7111-7130. doi: 10.7150/thno.45286. eCollection 2020. Theranostics. 2020. PMID: 32641982 Free PMC article.
-
Anterior expansion and posterior addition to the notochord mechanically coordinate zebrafish embryo axis elongation.Development. 2021 Sep 15;148(18):dev199459. doi: 10.1242/dev.199459. Epub 2021 Jul 21. Development. 2021. PMID: 34086031 Free PMC article.
-
DSTYK Enhances Chemoresistance in Triple-Negative Breast Cancer Cells.Cells. 2021 Dec 29;11(1):97. doi: 10.3390/cells11010097. Cells. 2021. PMID: 35011659 Free PMC article.
References
Publication types
MeSH terms
Substances
LinkOut - more resources
Full Text Sources
Medical
Molecular Biology Databases