NEDD9 Inhibition by miR-25-5p Activation Is Critically Involved in Co-Treatment of Melatonin- and Pterostilbene-Induced Apoptosis in Colorectal Cancer Cells
- PMID: 31671847
- PMCID: PMC6895813
- DOI: 10.3390/cancers11111684
NEDD9 Inhibition by miR-25-5p Activation Is Critically Involved in Co-Treatment of Melatonin- and Pterostilbene-Induced Apoptosis in Colorectal Cancer Cells
Abstract
The underlying interaction between melatonin (MLT) and daily fruit intake still remains unclear to date, despite multibiological effects of MLT. Herein, the apoptotic mechanism by co-treatment of MLT and pterostilbene (Ptero) contained mainly in grape and blueberries was elucidated in colorectal cancers (CRCs). MLT and Ptero co-treatment (MLT+Ptero) showed synergistic cytotoxicity compared with MLT or Ptero alone, reduced the number of colonies and Ki67 expression, and also increased terminal deoxynucleotidyl transferase dUTP nick end labeling- (TUNEL) positive cells and reactive oxygen species (ROS) production in CRCs. Consistently, MLT+Ptero cleaved caspase 3 and poly (ADP-ribose) polymerase (PARP), activated sex-determining region Y-Box10 (SOX10), and also attenuated the expression of Bcl-xL, neural precursor cell expressed developmentally downregulated protein 9 (NEDD9), and SOX9 in CRCs. Additionally, MLT+Ptero induced differentially expressed microRNAs (upregulation: miR-25-5p, miR-542-5p, miR-711, miR-4725-3p, and miR-4484; downregulation: miR-4504, miR-668-3p, miR-3121-5p, miR-195-3p, and miR-5194) in HT29 cells. Consistently, MLT +Ptero upregulated miR-25-5p at mRNA level and conversely NEDD9 overexpression or miR-25-5p inhibitor reversed the ability of MLT+Ptero to increase cytotoxicity, suppress colony formation, and cleave PARP in CRCs. Furthermore, immunofluorescence confirmed miR-25-5p inhibitor reversed the reduced fluorescence of NEDD9 and increased SOX10 by MLT+Ptero in HT29 cells. Taken together, our findings provided evidence that MLT+Ptero enhances apoptosis via miR-25-5p mediated NEDD9 inhibition in colon cancer cells as a potent strategy for colorectal cancer therapy.
Keywords: MLT; NEDD9; Ptero; apoptosis; miR-25-5p; synergy.
Conflict of interest statement
The authors declare no conflict of interest.
Figures
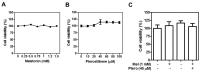
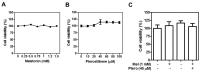
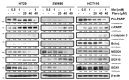
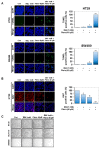
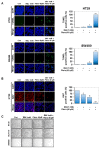
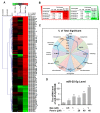
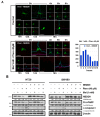
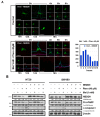
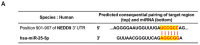
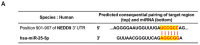
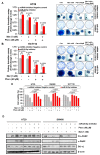
Similar articles
-
Melatonin Inhibits the Progression of Oral Squamous Cell Carcinoma via Inducing miR-25-5p Expression by Directly Targeting NEDD9.Front Oncol. 2020 Dec 2;10:543591. doi: 10.3389/fonc.2020.543591. eCollection 2020. Front Oncol. 2020. PMID: 33344223 Free PMC article.
-
Reactive oxygen species-mediated phosphorylation of p38 signaling is critically involved in apoptotic effect of Tanshinone I in colon cancer cells.Phytother Res. 2018 Oct;32(10):1975-1982. doi: 10.1002/ptr.6126. Epub 2018 Jun 7. Phytother Res. 2018. PMID: 29876988
-
Ursolic Acid Induces Apoptosis in Colorectal Cancer Cells Partially via Upregulation of MicroRNA-4500 and Inhibition of JAK2/STAT3 Phosphorylation.Int J Mol Sci. 2018 Dec 29;20(1):114. doi: 10.3390/ijms20010114. Int J Mol Sci. 2018. PMID: 30597956 Free PMC article.
-
High Glucose-Induced Apoptosis in Human Kidney Cells Was Alleviated by miR-15b-5p Mimics.Biol Pharm Bull. 2019 May 1;42(5):758-763. doi: 10.1248/bpb.b18-00951. Epub 2019 Mar 6. Biol Pharm Bull. 2019. PMID: 30842352
-
miR193a-5p Mediated ZNF746 and c-Myc Signaling Axis Is Critically Involved in Morusin Induced Apoptosis in Colorectal Cancer Cells.Cells. 2021 Aug 12;10(8):2065. doi: 10.3390/cells10082065. Cells. 2021. PMID: 34440834 Free PMC article.
Cited by
-
NEDD9 overexpression: Prognostic and guidance value in acute myeloid leukaemia.J Cell Mol Med. 2021 Oct;25(19):9331-9339. doi: 10.1111/jcmm.16870. Epub 2021 Aug 25. J Cell Mol Med. 2021. PMID: 34432355 Free PMC article.
-
Pterostilbene Enhances Cytotoxicity and Chemosensitivity in Human Pancreatic Cancer Cells.Biomolecules. 2020 May 4;10(5):709. doi: 10.3390/biom10050709. Biomolecules. 2020. PMID: 32375296 Free PMC article.
-
Crosstalk between noncoding RNAs and ferroptosis: new dawn for overcoming cancer progression.Cell Death Dis. 2020 Jul 24;11(7):580. doi: 10.1038/s41419-020-02772-8. Cell Death Dis. 2020. PMID: 32709863 Free PMC article. Review.
-
Circular RNA profiles and the potential involvement of down-expression of hsa_circ_0001360 in cutaneous squamous cell carcinogenesis.FEBS Open Bio. 2021 Apr;11(4):1209-1222. doi: 10.1002/2211-5463.13114. Epub 2021 Mar 11. FEBS Open Bio. 2021. PMID: 33569895 Free PMC article.
-
Role and Therapeutic Potential of Melatonin in Various Type of Cancers.Onco Targets Ther. 2021 Mar 18;14:2019-2052. doi: 10.2147/OTT.S298512. eCollection 2021. Onco Targets Ther. 2021. PMID: 33776451 Free PMC article. Review.
References
-
- Blask D.E., Wilson S.T., Zalatan F. Physiological MLT inhibition of human breast cancer cell growth in vitro: Evidence for a glutathione-mediated pathway. Cancer Res. 1997;57:1909–1914. - PubMed
Grants and funding
LinkOut - more resources
Full Text Sources
Research Materials