The molecular chaperone Hsp90α deficiency causes retinal degeneration by disrupting Golgi organization and vesicle transportation in photoreceptors
- PMID: 31408169
- PMCID: PMC7181719
- DOI: 10.1093/jmcb/mjz048
The molecular chaperone Hsp90α deficiency causes retinal degeneration by disrupting Golgi organization and vesicle transportation in photoreceptors
Abstract
Heat shock protein 90 (Hsp90) is an abundant molecular chaperone with two isoforms, Hsp90α and Hsp90β. Hsp90β deficiency causes embryonic lethality, whereas Hsp90α deficiency causes few abnormities except male sterility. In this paper, we reported that Hsp90α was exclusively expressed in the retina, testis, and brain. Its deficiency caused retinitis pigmentosa (RP), a disease leading to blindness. In Hsp90α-deficient mice, the retina was deteriorated and the outer segment of photoreceptor was deformed. Immunofluorescence staining and electron microscopic analysis revealed disintegrated Golgi and aberrant intersegmental vesicle transportation in Hsp90α-deficient photoreceptors. Proteomic analysis identified microtubule-associated protein 1B (MAP1B) as an Hsp90α-associated protein in photoreceptors. Hspα deficiency increased degradation of MAP1B by inducing its ubiquitination, causing α-tubulin deacetylation and microtubule destabilization. Furthermore, the treatment of wild-type mice with 17-DMAG, an Hsp90 inhibitor of geldanamycin derivative, induced the same retinal degeneration as Hsp90α deficiency. Taken together, the microtubule destabilization could be the underlying reason for Hsp90α deficiency-induced RP.
Keywords: Golgi disintegration; Hsp90α; MAP1B; acetylated α-tubulin; cytoskeleton; microtubule; retinitis pigmentosa; vesicle transportation.
© The Author(s) (2019). Published by Oxford University Press on behalf of Journal of Molecular Cell Biology, IBCB, SIBS, CAS.
Figures
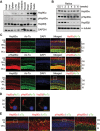
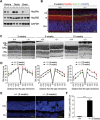
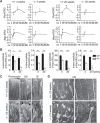
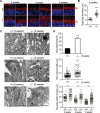
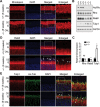
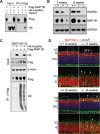
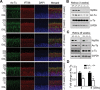
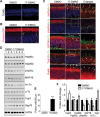
Similar articles
-
The molecular chaperone Hsp90 maintains Golgi organization and vesicular trafficking by regulating microtubule stability.J Mol Cell Biol. 2020 Jul 3;12(6):448-461. doi: 10.1093/jmcb/mjz093. J Mol Cell Biol. 2020. PMID: 31560394 Free PMC article.
-
HSP90α is needed for the survival of rod photoreceptors and regulates the expression of rod PDE6 subunits.J Biol Chem. 2023 Jun;299(6):104809. doi: 10.1016/j.jbc.2023.104809. Epub 2023 May 11. J Biol Chem. 2023. PMID: 37172722 Free PMC article.
-
The retinitis pigmentosa protein RP2 links pericentriolar vesicle transport between the Golgi and the primary cilium.Hum Mol Genet. 2010 Apr 1;19(7):1358-67. doi: 10.1093/hmg/ddq012. Epub 2010 Jan 27. Hum Mol Genet. 2010. PMID: 20106869
-
The Distinct Assignments for Hsp90α and Hsp90β: More Than Skin Deep.Cells. 2023 Jan 11;12(2):277. doi: 10.3390/cells12020277. Cells. 2023. PMID: 36672211 Free PMC article. Review.
-
The Role of Hsp90 in Retinal Proteostasis and Disease.Biomolecules. 2022 Jul 12;12(7):978. doi: 10.3390/biom12070978. Biomolecules. 2022. PMID: 35883534 Free PMC article. Review.
Cited by
-
Extracellular heat shock protein 90 alpha (eHsp90α)'s role in cancer progression and the development of therapeutic strategies.Eur J Med Chem. 2024 Nov 5;277:116736. doi: 10.1016/j.ejmech.2024.116736. Epub 2024 Aug 2. Eur J Med Chem. 2024. PMID: 39126794 Review.
-
The molecular chaperone Hsp90 maintains Golgi organization and vesicular trafficking by regulating microtubule stability.J Mol Cell Biol. 2020 Jul 3;12(6):448-461. doi: 10.1093/jmcb/mjz093. J Mol Cell Biol. 2020. PMID: 31560394 Free PMC article.
-
Predictive Modeling of MAFLD Based on Hsp90α and the Therapeutic Application of Teprenone in a Diet-Induced Mouse Model.Front Endocrinol (Lausanne). 2021 Sep 30;12:743202. doi: 10.3389/fendo.2021.743202. eCollection 2021. Front Endocrinol (Lausanne). 2021. PMID: 34659125 Free PMC article.
-
Divergent Effects of HSP70 Overexpression in Photoreceptors During Inherited Retinal Degeneration.Invest Ophthalmol Vis Sci. 2020 Oct 1;61(12):25. doi: 10.1167/iovs.61.12.25. Invest Ophthalmol Vis Sci. 2020. PMID: 33107904 Free PMC article.
-
Translational reprogramming in response to accumulating stressors ensures critical threshold levels of Hsp90 for mammalian life.Nat Commun. 2022 Oct 21;13(1):6271. doi: 10.1038/s41467-022-33916-3. Nat Commun. 2022. PMID: 36270993 Free PMC article.
References
-
- Besharse J.C., and Horst C.J. (1990). The photoreceptor connecting cilium A model for the transition zone In: Bloodgood, R.A. (ed.). Ciliary and Flagellar Membranes. Boston, MA: Springer, 389–417.
Publication types
MeSH terms
Substances
LinkOut - more resources
Full Text Sources
Molecular Biology Databases