Senolytic therapy alleviates Aβ-associated oligodendrocyte progenitor cell senescence and cognitive deficits in an Alzheimer's disease model
- PMID: 30936558
- PMCID: PMC6605052
- DOI: 10.1038/s41593-019-0372-9
Senolytic therapy alleviates Aβ-associated oligodendrocyte progenitor cell senescence and cognitive deficits in an Alzheimer's disease model
Abstract
Neuritic plaques, a pathological hallmark in Alzheimer's disease (AD) brains, comprise extracellular aggregates of amyloid-beta (Aβ) peptide and degenerating neurites that accumulate autolysosomes. We found that, in the brains of patients with AD and in AD mouse models, Aβ plaque-associated Olig2- and NG2-expressing oligodendrocyte progenitor cells (OPCs), but not astrocytes, microglia, or oligodendrocytes, exhibit a senescence-like phenotype characterized by the upregulation of p21/CDKN1A, p16/INK4/CDKN2A proteins, and senescence-associated β-galactosidase activity. Molecular interrogation of the Aβ plaque environment revealed elevated levels of transcripts encoding proteins involved in OPC function, replicative senescence, and inflammation. Direct exposure of cultured OPCs to aggregating Aβ triggered cell senescence. Senolytic treatment of AD mice selectively removed senescent cells from the plaque environment, reduced neuroinflammation, lessened Aβ load, and ameliorated cognitive deficits. Our findings suggest a role for Aβ-induced OPC cell senescence in neuroinflammation and cognitive deficits in AD, and a potential therapeutic benefit of senolytic treatments.
Conflict of interest statement
Competing interests
The authors declare no competing interests.
Figures
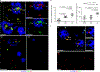
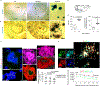
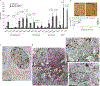
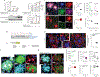
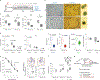
Comment in
-
Senescent glia spell trouble in Alzheimer's disease.Nat Neurosci. 2019 May;22(5):683-684. doi: 10.1038/s41593-019-0395-2. Nat Neurosci. 2019. PMID: 31024116 No abstract available.
Similar articles
-
Disruption of oligodendrocyte progenitor cells is an early sign of pathology in the triple transgenic mouse model of Alzheimer's disease.Neurobiol Aging. 2020 Oct;94:130-139. doi: 10.1016/j.neurobiolaging.2020.05.016. Epub 2020 Jun 7. Neurobiol Aging. 2020. PMID: 32619874 Free PMC article.
-
Amyloid β Protein Aggravates Neuronal Senescence and Cognitive Deficits in 5XFAD Mouse Model of Alzheimer's Disease.Chin Med J (Engl). 2016 Aug 5;129(15):1835-44. doi: 10.4103/0366-6999.186646. Chin Med J (Engl). 2016. PMID: 27453234 Free PMC article.
-
Fibrillar Aβ triggers microglial proteome alterations and dysfunction in Alzheimer mouse models.Elife. 2020 Jun 8;9:e54083. doi: 10.7554/eLife.54083. Elife. 2020. PMID: 32510331 Free PMC article.
-
Oligodendrocyte progenitor cells in Alzheimer's disease: from physiology to pathology.Transl Neurodegener. 2023 Nov 14;12(1):52. doi: 10.1186/s40035-023-00385-7. Transl Neurodegener. 2023. PMID: 37964328 Free PMC article. Review.
-
Aging, Cellular Senescence, and Alzheimer's Disease.Int J Mol Sci. 2022 Feb 11;23(4):1989. doi: 10.3390/ijms23041989. Int J Mol Sci. 2022. PMID: 35216123 Free PMC article. Review.
Cited by
-
White matter and neurological disorders.Arch Pharm Res. 2020 Sep;43(9):920-931. doi: 10.1007/s12272-020-01270-x. Epub 2020 Sep 25. Arch Pharm Res. 2020. PMID: 32975736 Review.
-
Alzheimer's Amyloid-β Accelerates Cell Senescence and Suppresses the SIRT1/NRF2 Pathway in Human Microglial Cells.Oxid Med Cell Longev. 2022 Aug 17;2022:3086010. doi: 10.1155/2022/3086010. eCollection 2022. Oxid Med Cell Longev. 2022. PMID: 36035216 Free PMC article.
-
P53 aggregation, interactions with tau, and impaired DNA damage response in Alzheimer's disease.Acta Neuropathol Commun. 2020 Aug 10;8(1):132. doi: 10.1186/s40478-020-01012-6. Acta Neuropathol Commun. 2020. PMID: 32778161 Free PMC article.
-
EED-mediated histone methylation is critical for CNS myelination and remyelination by inhibiting WNT, BMP, and senescence pathways.Sci Adv. 2020 Aug 12;6(33):eaaz6477. doi: 10.1126/sciadv.aaz6477. eCollection 2020 Aug. Sci Adv. 2020. PMID: 32851157 Free PMC article.
-
Replicative senescence dictates the emergence of disease-associated microglia and contributes to Aβ pathology.Cell Rep. 2021 Jun 8;35(10):109228. doi: 10.1016/j.celrep.2021.109228. Cell Rep. 2021. PMID: 34107254 Free PMC article.
References
-
- Wyss-Coray T Inflammation in Alzheimer disease: driving force, bystander or beneficial response? Nat. Med 12, 1005–1015 (2006). - PubMed
-
- Nixon RA The role of autophagy in neurodegenerative disease. Nat. Med 19, 983–997 (2013). - PubMed
-
- Scheltens P et al. Alzheimer’s disease. Lancet 388, 505–517 (2016). - PubMed
-
- Muñoz-Espín D & Serrano M Cellular senescence: from physiology to pathology. Nat. Rev. Mol. Cell Biol 15, 482–496 (2014). - PubMed
Publication types
MeSH terms
Substances
Grants and funding
LinkOut - more resources
Full Text Sources
Other Literature Sources
Medical
Molecular Biology Databases
Research Materials
Miscellaneous