Traumatic injury induces stress granule formation and enhances motor dysfunctions in ALS/FTD models
- PMID: 29432563
- PMCID: PMC6455923
- DOI: 10.1093/hmg/ddy047
Traumatic injury induces stress granule formation and enhances motor dysfunctions in ALS/FTD models
Abstract
Traumatic brain injury (TBI) has been predicted to be a predisposing factor for amyotrophic lateral sclerosis (ALS) and other neurological disorders. Despite the importance of TBI in ALS progression, the underlying cellular and molecular mechanisms are still an enigma. Here, we examined the contribution of TBI as an extrinsic factor and investigated whether TBI influences the susceptibility of developing neurodegenerative symptoms. To evaluate the effects of TBI in vivo, we applied mild to severe trauma to Drosophila and found that TBI leads to the induction of stress granules (SGs) in the brain. The degree of SGs induction directly correlates with the level of trauma. Furthermore, we observed that the level of mortality is directly proportional to the number of traumatic hits. Interestingly, trauma-induced SGs are ubiquitin, p62 and TDP-43 positive, and persistently remain over time suggesting that SGs might be aggregates and exert toxicity in our fly models. Intriguingly, TBI on animals expressing ALS-linked genes increased mortality and locomotion dysfunction suggesting that mild trauma might aggravate neurodegenerative symptoms associated with ALS. Furthermore, we found elevated levels of high molecular weight ubiquitinated proteins and p62 in animals expressing ALS-causing genes with TBI, suggesting that TBI may lead to the defects in protein degradation pathways. Finally, we observed that genetic and pharmacological induction of autophagy enhanced the clearance of SGs and promoted survival of flies in vivo. Together, our study demonstrates that trauma can induce SG formation in vivo and might enhance neurodegenerative phenotypes in the fly models of ALS.
Figures
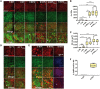
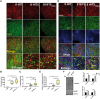
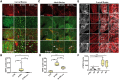
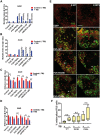
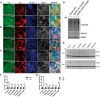
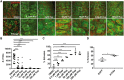
Similar articles
-
Traumatic injury compromises nucleocytoplasmic transport and leads to TDP-43 pathology.Elife. 2021 May 26;10:e67587. doi: 10.7554/eLife.67587. Elife. 2021. PMID: 34060470 Free PMC article.
-
Reversible induction of TDP-43 granules in cortical neurons after traumatic injury.Exp Neurol. 2018 Jan;299(Pt A):15-25. doi: 10.1016/j.expneurol.2017.09.011. Epub 2017 Sep 21. Exp Neurol. 2018. PMID: 28941811
-
The ALS-associated proteins FUS and TDP-43 function together to affect Drosophila locomotion and life span.J Clin Invest. 2011 Oct;121(10):4118-26. doi: 10.1172/JCI57883. Epub 2011 Sep 1. J Clin Invest. 2011. PMID: 21881207 Free PMC article.
-
C9orf72 ALS-FTD: recent evidence for dysregulation of the autophagy-lysosome pathway at multiple levels.Autophagy. 2021 Nov;17(11):3306-3322. doi: 10.1080/15548627.2021.1872189. Epub 2021 Feb 26. Autophagy. 2021. PMID: 33632058 Free PMC article. Review.
-
Stress granule mediated protein aggregation and underlying gene defects in the FTD-ALS spectrum.Neurobiol Dis. 2020 Feb;134:104639. doi: 10.1016/j.nbd.2019.104639. Epub 2019 Oct 15. Neurobiol Dis. 2020. PMID: 31626953 Review.
Cited by
-
Stress granule assembly in vivo is deficient in the CNS of mutant TDP-43 ALS mice.Hum Mol Genet. 2023 Jan 6;32(2):319-332. doi: 10.1093/hmg/ddac206. Hum Mol Genet. 2023. PMID: 35994036 Free PMC article.
-
Synergistic effects of brain injury and aging: common mechanisms of proteostatic dysfunction.Trends Neurosci. 2021 Sep;44(9):728-740. doi: 10.1016/j.tins.2021.06.003. Epub 2021 Jul 20. Trends Neurosci. 2021. PMID: 34301397 Free PMC article. Review.
-
SQSTM1/p62 and Hepatic Mallory-Denk Body Formation in Alcohol-Associated Liver Disease.Am J Pathol. 2023 Oct;193(10):1415-1426. doi: 10.1016/j.ajpath.2023.02.015. Epub 2023 Mar 9. Am J Pathol. 2023. PMID: 36906265 Free PMC article. Review.
-
Phenotypic Suppression of ALS/FTD-Associated Neurodegeneration Highlights Mechanisms of Dysfunction.J Neurosci. 2019 Oct 16;39(42):8217-8224. doi: 10.1523/JNEUROSCI.1159-19.2019. J Neurosci. 2019. PMID: 31619490 Free PMC article. Review.
-
Drosha-dependent microRNAs modulate FUS-mediated neurodegeneration in vivo.Nucleic Acids Res. 2023 Nov 10;51(20):11258-11276. doi: 10.1093/nar/gkad774. Nucleic Acids Res. 2023. PMID: 37791873 Free PMC article.
References
-
- Kuusisto E., Salminen A., Alafuzoff I. (2001) Ubiquitin-binding protein p62 is present in neuronal and glial inclusions in human tauopathies and synucleinopathies. Neuroreport, 12, 2085–2090. - PubMed
-
- Terni B., Rey M.J., Boluda S., Torrejon-Escribano B., Sabate M.P., Calopa M., van Leeuwen F.W., Ferrer I. (2007) Mutant ubiquitin and p62 immunoreactivity in cases of combined multiple system atrophy and Alzheimer's disease. Acta Neuropathol., 113, 403–416. - PubMed
-
- Salminen A., Kaarniranta K., Haapasalo A., Hiltunen M., Soininen H., Alafuzoff I. (2012) Emerging role of p62/sequestosome-1 in the pathogenesis of Alzheimer's disease. Prog. Neurobiol., 96, 87–95. - PubMed
-
- Kuusisto E., Salminen A., Alafuzoff I. (2002) Early accumulation of p62 in neurofibrillary tangles in Alzheimer's disease: possible role in tangle formation. Neuropathol. Appl. Neurobiol., 28, 228–237. - PubMed
-
- Arai T., Hasegawa M., Akiyama H., Ikeda K., Nonaka T., Mori H., Mann D., Tsuchiya K., Yoshida M., Hashizume Y. (2006) TDP-43 is a component of ubiquitin-positive tau-negative inclusions in frontotemporal lobar degeneration and amyotrophic lateral sclerosis. Biochem. Biophys. Res. Commun., 351, 602–611. - PubMed
Publication types
MeSH terms
Substances
Supplementary concepts
Grants and funding
LinkOut - more resources
Full Text Sources
Other Literature Sources
Medical
Molecular Biology Databases
Miscellaneous