Modulating the Tumor Microenvironment to Enhance Tumor Nanomedicine Delivery
- PMID: 29311946
- PMCID: PMC5744178
- DOI: 10.3389/fphar.2017.00952
Modulating the Tumor Microenvironment to Enhance Tumor Nanomedicine Delivery
Abstract
Nanomedicines including liposomes, micelles, and nanoparticles based on the enhanced permeability and retention (EPR) effect have become the mainstream for tumor treatment owing to their superiority over conventional anticancer agents. Advanced design of nanomedicine including active targeting nanomedicine, tumor-responsive nanomedicine, and optimization of physicochemical properties to enable highly effective delivery of nanomedicine to tumors has further improved their therapeutic benefits. However, these strategies still could not conquer the delivery barriers of a tumor microenvironment such as heterogeneous blood flow, dense extracellular matrix, abundant stroma cells, and high interstitial fluid pressure, which severely impaired vascular transport of nanomedicines, hindered their effective extravasation, and impeded their interstitial transport to realize uniform distribution inside tumors. Therefore, modulation of tumor microenvironment has now emerged as an important strategy to improve nanomedicine delivery to tumors. Here, we review the existing strategies and approaches for tumor microenvironment modulation to improve tumor perfusion for helping more nanomedicines to reach the tumor site, to facilitate nanomedicine extravasation for enhancing transvascular transport, and to improve interstitial transport for optimizing the distribution of nanomedicines. These strategies may provide an avenue for the development of new combination chemotherapeutic regimens and reassessment of previously suboptimal agents.
Keywords: extracellular matrix; interstitial fluid pressure; nanomedicine; tumor microenvironment; tumor nanomedicine delivery; tumor perfusion.
Figures
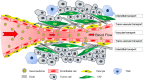
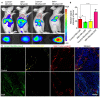
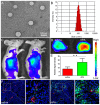
Similar articles
-
Strategies to improve tumor penetration of nanomedicines through nanoparticle design.Wiley Interdiscip Rev Nanomed Nanobiotechnol. 2019 Jan;11(1):e1519. doi: 10.1002/wnan.1519. Epub 2018 Apr 16. Wiley Interdiscip Rev Nanomed Nanobiotechnol. 2019. PMID: 29659166 Review.
-
Paclitaxel-loaded micelles enhance transvascular permeability and retention of nanomedicines in tumors.Int J Pharm. 2015 Feb 20;479(2):399-407. doi: 10.1016/j.ijpharm.2015.01.009. Epub 2015 Jan 8. Int J Pharm. 2015. PMID: 25578367
-
Current Approaches for Improving Intratumoral Accumulation and Distribution of Nanomedicines.Theranostics. 2015 Jun 8;5(9):1007-20. doi: 10.7150/thno.11742. eCollection 2015. Theranostics. 2015. PMID: 26155316 Free PMC article. Review.
-
Tumor extravasation and infiltration as barriers of nanomedicine for high efficacy: The current status and transcytosis strategy.Biomaterials. 2020 May;240:119902. doi: 10.1016/j.biomaterials.2020.119902. Epub 2020 Feb 18. Biomaterials. 2020. PMID: 32105817 Review.
-
Optimization of the tumor microenvironment and nanomedicine properties simultaneously to improve tumor therapy.Oncotarget. 2016 Sep 20;7(38):62607-62618. doi: 10.18632/oncotarget.11546. Oncotarget. 2016. PMID: 27566585 Free PMC article.
Cited by
-
Nanoscale platform for delivery of active IRINOX to combat pancreatic cancer.J Control Release. 2021 Feb 10;330:1229-1243. doi: 10.1016/j.jconrel.2020.11.029. Epub 2020 Nov 18. J Control Release. 2021. PMID: 33217475 Free PMC article.
-
Nanocarriers as a Delivery Platform for Anticancer Treatment: Biological Limits and Perspectives in B-Cell Malignancies.Pharmaceutics. 2022 Sep 17;14(9):1965. doi: 10.3390/pharmaceutics14091965. Pharmaceutics. 2022. PMID: 36145713 Free PMC article. Review.
-
Recent advances and challenges of repurposing nanoparticle-based drug delivery systems to enhance cancer immunotherapy.Theranostics. 2019 Oct 16;9(25):7906-7923. doi: 10.7150/thno.38425. eCollection 2019. Theranostics. 2019. PMID: 31695807 Free PMC article. Review.
-
Approaches to Improve EPR-Based Drug Delivery for Cancer Therapy and Diagnosis.J Pers Med. 2023 Feb 23;13(3):389. doi: 10.3390/jpm13030389. J Pers Med. 2023. PMID: 36983571 Free PMC article. Review.
-
An Overview of Antibody Conjugated Polymeric Nanoparticles for Breast Cancer Therapy.Pharmaceutics. 2020 Aug 25;12(9):802. doi: 10.3390/pharmaceutics12090802. Pharmaceutics. 2020. PMID: 32854255 Free PMC article. Review.
References
-
- Boucher Y., Baxter L. T., Jain R. K. (1990). Interstitial pressure gradients in tissue-isolated and subcutaneous tumors: implications for therapy. Cancer Res. 50, 4478–4484. - PubMed
Publication types
LinkOut - more resources
Full Text Sources
Other Literature Sources