Short DNA Hairpins Compromise Recombinant Adeno-Associated Virus Genome Homogeneity
- PMID: 28462820
- PMCID: PMC5474962
- DOI: 10.1016/j.ymthe.2017.03.028
Short DNA Hairpins Compromise Recombinant Adeno-Associated Virus Genome Homogeneity
Abstract
Short hairpin (sh)RNAs delivered by recombinant adeno-associated viruses (rAAVs) are valuable tools to study gene function in vivo and a promising gene therapy platform. Our data show that incorporation of shRNA transgenes into rAAV constructs reduces vector yield and produces a population of truncated and defective genomes. We demonstrate that sequences with hairpins or hairpin-like structures drive the generation of truncated AAV genomes through a polymerase redirection mechanism during viral genome replication. Our findings reveal the importance of genomic secondary structure when optimizing viral vector designs. We also discovered that shDNAs could be adapted to act as surrogate mutant inverted terminal repeats (mTRs), sequences that were previously thought to be required for functional self-complementary AAV vectors. The use of shDNAs as artificial mTRs opens the door to engineering a new generation of AAV vectors with improved potency, genetic stability, and safety for both preclinical studies and human gene therapy.
Keywords: adeno-associated virus; genome homogeneity; replication template switching; self-complementary AAV; short hairpin DNA; short hairpin RNA; viral genome truncation.
Published by Elsevier Inc.
Figures
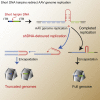
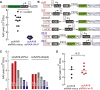
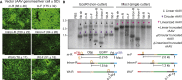
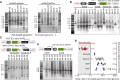
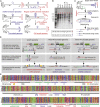
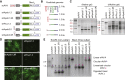
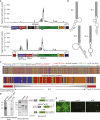
Similar articles
-
Rescue and replication of adeno-associated virus type 2 as well as vector DNA sequences from recombinant plasmids containing deletions in the viral inverted terminal repeats: selective encapsidation of viral genomes in progeny virions.J Virol. 1996 Mar;70(3):1668-77. doi: 10.1128/JVI.70.3.1668-1677.1996. J Virol. 1996. PMID: 8627687 Free PMC article.
-
Adeno-Associated Virus Serotype-Specific Inverted Terminal Repeat Sequence Role in Vector Transgene Expression.Hum Gene Ther. 2020 Feb;31(3-4):151-162. doi: 10.1089/hum.2019.274. Hum Gene Ther. 2020. PMID: 31914802 Free PMC article.
-
Integrating adenovirus-adeno-associated virus hybrid vectors devoid of all viral genes.J Virol. 1999 Nov;73(11):9314-24. doi: 10.1128/JVI.73.11.9314-9324.1999. J Virol. 1999. PMID: 10516040 Free PMC article.
-
Expressing Transgenes That Exceed the Packaging Capacity of Adeno-Associated Virus Capsids.Hum Gene Ther Methods. 2016 Feb;27(1):1-12. doi: 10.1089/hgtb.2015.140. Hum Gene Ther Methods. 2016. PMID: 26757051 Free PMC article. Review.
-
Adeno-associated viral vectors for gene transfer and gene therapy.Biol Chem. 1999 Jun;380(6):613-22. doi: 10.1515/BC.1999.078. Biol Chem. 1999. PMID: 10430026 Review.
Cited by
-
Regulation of sclerostin by the SIRT1 stabilization pathway in osteocytes.Cell Death Differ. 2022 Aug;29(8):1625-1638. doi: 10.1038/s41418-022-00952-x. Epub 2022 Feb 15. Cell Death Differ. 2022. PMID: 35169297 Free PMC article.
-
Engineering a targeted and safe bone anabolic gene therapy to treat osteoporosis in alveolar bone loss.Mol Ther. 2024 Sep 4;32(9):3080-3100. doi: 10.1016/j.ymthe.2024.06.036. Epub 2024 Jun 26. Mol Ther. 2024. PMID: 38937970 Free PMC article.
-
AAV-delivered suppressor tRNA overcomes a nonsense mutation in mice.Nature. 2022 Apr;604(7905):343-348. doi: 10.1038/s41586-022-04533-3. Epub 2022 Mar 23. Nature. 2022. PMID: 35322228 Free PMC article.
-
Ex vivo and in vivo suppression of SARS-CoV-2 with combinatorial AAV/RNAi expression vectors.Mol Ther. 2022 May 4;30(5):2005-2023. doi: 10.1016/j.ymthe.2022.01.024. Epub 2022 Jan 14. Mol Ther. 2022. PMID: 35038579 Free PMC article.
-
Gene targeting as a therapeutic avenue in diseases mediated by the complement alternative pathway.Immunol Rev. 2023 Jan;313(1):402-419. doi: 10.1111/imr.13149. Epub 2022 Nov 12. Immunol Rev. 2023. PMID: 36369963 Free PMC article. Review.
References
-
- Handa H., Carter B.J. Adeno-associated virus DNA replication complexes in herpes simplex virus or adenovirus-infected cells. J. Biol. Chem. 1979;254:6603–6610. - PubMed
-
- de la Maza L.M., Carter B.J. Molecular structure of adeno-associated virus variant DNA. J. Biol. Chem. 1980;255:3194–3203. - PubMed
Publication types
MeSH terms
Substances
Grants and funding
LinkOut - more resources
Full Text Sources
Other Literature Sources