Structural and Functional Analysis of a β2-Adrenergic Receptor Complex with GRK5
- PMID: 28431242
- PMCID: PMC5526774
- DOI: 10.1016/j.cell.2017.03.047
Structural and Functional Analysis of a β2-Adrenergic Receptor Complex with GRK5
Abstract
The phosphorylation of agonist-occupied G-protein-coupled receptors (GPCRs) by GPCR kinases (GRKs) functions to turn off G-protein signaling and turn on arrestin-mediated signaling. While a structural understanding of GPCR/G-protein and GPCR/arrestin complexes has emerged in recent years, the molecular architecture of a GPCR/GRK complex remains poorly defined. We used a comprehensive integrated approach of cross-linking, hydrogen-deuterium exchange mass spectrometry (MS), electron microscopy, mutagenesis, molecular dynamics simulations, and computational docking to analyze GRK5 interaction with the β2-adrenergic receptor (β2AR). These studies revealed a dynamic mechanism of complex formation that involves large conformational changes in the GRK5 RH/catalytic domain interface upon receptor binding. These changes facilitate contacts between intracellular loops 2 and 3 and the C terminus of the β2AR with the GRK5 RH bundle subdomain, membrane-binding surface, and kinase catalytic cleft, respectively. These studies significantly contribute to our understanding of the mechanism by which GRKs regulate the function of activated GPCRs. PAPERCLIP.
Keywords: G-protein-coupled receptor; G-protein-coupled receptor kinases; cross-linking; mass spectrometry; molecular dynamics; phosphorylation; β(2)-adrenergic receptor.
Copyright © 2017 Elsevier Inc. All rights reserved.
Figures
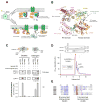
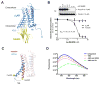
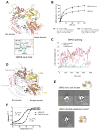
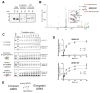
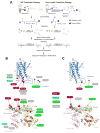
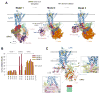
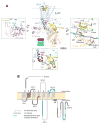
Similar articles
-
Structure of a GRK5-Calmodulin Complex Reveals Molecular Mechanism of GRK Activation and Substrate Targeting.Mol Cell. 2021 Jan 21;81(2):323-339.e11. doi: 10.1016/j.molcel.2020.11.026. Epub 2020 Dec 14. Mol Cell. 2021. PMID: 33321095 Free PMC article.
-
Antagonistic Roles of GRK2 and GRK5 in Cardiac Aldosterone Signaling Reveal GRK5-Mediated Cardioprotection via Mineralocorticoid Receptor Inhibition.Int J Mol Sci. 2020 Apr 20;21(8):2868. doi: 10.3390/ijms21082868. Int J Mol Sci. 2020. PMID: 32326036 Free PMC article.
-
Differential regulation of β2-adrenoceptor and adenosine A2B receptor signalling by GRK and arrestin proteins in arterial smooth muscle.Cell Signal. 2018 Nov;51:86-98. doi: 10.1016/j.cellsig.2018.07.013. Epub 2018 Jul 31. Cell Signal. 2018. PMID: 30075183
-
Structural features of β2 adrenergic receptor: crystal structures and beyond.Mol Cells. 2015;38(2):105-11. doi: 10.14348/molcells.2015.2301. Epub 2014 Dec 24. Mol Cells. 2015. PMID: 25537861 Free PMC article. Review.
-
The power of mass spectrometry in structural characterization of GPCR signaling.J Recept Signal Transduct Res. 2015 Jun;35(3):213-9. doi: 10.3109/10799893.2015.1072979. Epub 2015 Oct 12. J Recept Signal Transduct Res. 2015. PMID: 26459735 Review.
Cited by
-
Modeling the complete chemokine-receptor interaction.Methods Cell Biol. 2019;149:289-314. doi: 10.1016/bs.mcb.2018.09.005. Epub 2018 Nov 1. Methods Cell Biol. 2019. PMID: 30616825 Free PMC article.
-
Conformational dynamics of auto-inhibition in the ER calcium sensor STIM1.Elife. 2021 Nov 3;10:e66194. doi: 10.7554/eLife.66194. Elife. 2021. PMID: 34730514 Free PMC article.
-
GPCRs and Signal Transducers: Interaction Stoichiometry.Trends Pharmacol Sci. 2018 Jul;39(7):672-684. doi: 10.1016/j.tips.2018.04.002. Epub 2018 May 5. Trends Pharmacol Sci. 2018. PMID: 29739625 Free PMC article. Review.
-
Function and dynamics of the intrinsically disordered carboxyl terminus of β2 adrenergic receptor.Nat Commun. 2023 Apr 10;14(1):2005. doi: 10.1038/s41467-023-37233-1. Nat Commun. 2023. PMID: 37037825 Free PMC article.
-
Structure of a GRK5-Calmodulin Complex Reveals Molecular Mechanism of GRK Activation and Substrate Targeting.Mol Cell. 2021 Jan 21;81(2):323-339.e11. doi: 10.1016/j.molcel.2020.11.026. Epub 2020 Dec 14. Mol Cell. 2021. PMID: 33321095 Free PMC article.
References
-
- Case DA, Betz RM, Cerutti DS, Cheatham TE, III , Darden TA, Duke RE, Giese TJ, Gohlke H, Goetz AW, Homeyer N, et al. AMBER 2016. University of California; San Francisco: 2016.
-
- Cox J, Mann M. MaxQuant enables high peptide identification rates, individualized p. p.b.-range mass accuracies and proteome-wide protein quantification. Nat Biotechnol. 2008;26:1367–1372. - PubMed
Publication types
MeSH terms
Substances
Grants and funding
LinkOut - more resources
Full Text Sources
Other Literature Sources
Research Materials