Gambogic acid identifies an isoform-specific druggable pocket in the middle domain of Hsp90β
- PMID: 27466407
- PMCID: PMC4995996
- DOI: 10.1073/pnas.1606655113
Gambogic acid identifies an isoform-specific druggable pocket in the middle domain of Hsp90β
Abstract
Because of their importance in maintaining protein homeostasis, molecular chaperones, including heat-shock protein 90 (Hsp90), represent attractive drug targets. Although a number of Hsp90 inhibitors are in preclinical/clinical development, none strongly differentiate between constitutively expressed Hsp90β and stress-induced Hsp90α, the two cytosolic paralogs of this molecular chaperone. Thus, the importance of inhibiting one or the other paralog in different disease states remains unknown. We show that the natural product, gambogic acid (GBA), binds selectively to a site in the middle domain of Hsp90β, identifying GBA as an Hsp90β-specific Hsp90 inhibitor. Furthermore, using computational and medicinal chemistry, we identified a GBA analog, referred to as DAP-19, which binds potently and selectively to Hsp90β. Because of its unprecedented selectivity for Hsp90β among all Hsp90 paralogs, GBA thus provides a new chemical tool to study the unique biological role of this abundantly expressed molecular chaperone in health and disease.
Keywords: caged xanthone; heat-shock protein 90; isoform-specific inhibitor; molecular chaperone; molecular docking.
Conflict of interest statement
The authors declare no conflict of interest.
Figures
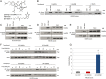
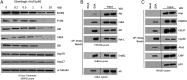
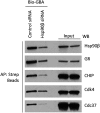
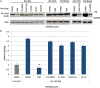
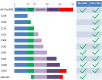
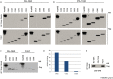
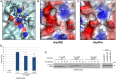
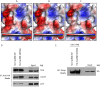
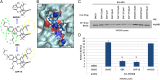
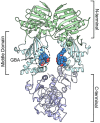
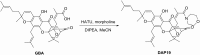
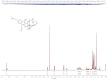
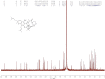
Similar articles
-
Identification of Isoform-Selective Ligands for the Middle Domain of Heat Shock Protein 90 (Hsp90).Int J Mol Sci. 2019 Oct 26;20(21):5333. doi: 10.3390/ijms20215333. Int J Mol Sci. 2019. PMID: 31717777 Free PMC article.
-
Client Proteins and Small Molecule Inhibitors Display Distinct Binding Preferences for Constitutive and Stress-Induced HSP90 Isoforms and Their Conformationally Restricted Mutants.PLoS One. 2015 Oct 30;10(10):e0141786. doi: 10.1371/journal.pone.0141786. eCollection 2015. PLoS One. 2015. PMID: 26517842 Free PMC article.
-
Expressed as the sole Hsp90 of yeast, the alpha and beta isoforms of human Hsp90 differ with regard to their capacities for activation of certain client proteins, whereas only Hsp90beta generates sensitivity to the Hsp90 inhibitor radicicol.FEBS J. 2007 Sep;274(17):4453-63. doi: 10.1111/j.1742-4658.2007.05974.x. Epub 2007 Aug 6. FEBS J. 2007. PMID: 17681020
-
Cytosolic Hsp90 Isoform-Specific Functions and Clinical Significance.Biomolecules. 2022 Aug 23;12(9):1166. doi: 10.3390/biom12091166. Biomolecules. 2022. PMID: 36139005 Free PMC article. Review.
-
The Distinct Assignments for Hsp90α and Hsp90β: More Than Skin Deep.Cells. 2023 Jan 11;12(2):277. doi: 10.3390/cells12020277. Cells. 2023. PMID: 36672211 Free PMC article. Review.
Cited by
-
Localized disruption of redox homeostasis boosting ferroptosis of tumor by hydrogel delivery system.Mater Today Bio. 2021 Nov 2;12:100154. doi: 10.1016/j.mtbio.2021.100154. eCollection 2021 Sep. Mater Today Bio. 2021. PMID: 34778741 Free PMC article.
-
Development of a High-throughput NanoBRET Screening Platform to Identify Modulators of the RAS/RAF Interaction.Mol Cancer Ther. 2021 Sep;20(9):1743-1754. doi: 10.1158/1535-7163.MCT-21-0175. Epub 2021 Jun 22. Mol Cancer Ther. 2021. PMID: 34158349 Free PMC article.
-
Methods to validate Hsp90 inhibitor specificity, to identify off-target effects, and to rethink approaches for further clinical development.Cell Stress Chaperones. 2018 Jul;23(4):467-482. doi: 10.1007/s12192-018-0877-2. Epub 2018 Feb 1. Cell Stress Chaperones. 2018. PMID: 29392504 Free PMC article. Review.
-
Gambogic acid: Multi-gram scale isolation, stereochemical erosion toward epi-gambogic acid and biological profile.Front Nat Prod. 2022;1:1018765. doi: 10.3389/fntpr.2022.1018765. Front Nat Prod. 2022. PMID: 39211297 Free PMC article.
-
Identification of Isoform-Selective Ligands for the Middle Domain of Heat Shock Protein 90 (Hsp90).Int J Mol Sci. 2019 Oct 26;20(21):5333. doi: 10.3390/ijms20215333. Int J Mol Sci. 2019. PMID: 31717777 Free PMC article.
References
-
- Taipale M, Jarosz DF, Lindquist S. HSP90 at the hub of protein homeostasis: Emerging mechanistic insights. Nat Rev Mol Cell Biol. 2010;11(7):515–528. - PubMed
-
- Zhao YH, et al. Upregulation of lactate dehydrogenase A by ErbB2 through heat shock factor 1 promotes breast cancer cell glycolysis and growth. Oncogene. 2009;28(42):3689–3701. - PubMed
-
- Minet E, et al. Hypoxia-induced activation of HIF-1: Role of HIF-1α-Hsp90 interaction. FEBS Lett. 1999;460(2):251–256. - PubMed
Publication types
MeSH terms
Substances
Grants and funding
LinkOut - more resources
Full Text Sources
Other Literature Sources
Miscellaneous