A viral kinase mimics S6 kinase to enhance cell proliferation
- PMID: 27342859
- PMCID: PMC4948314
- DOI: 10.1073/pnas.1600587113
A viral kinase mimics S6 kinase to enhance cell proliferation
Abstract
Viruses depend upon the host cell for manufacturing components of progeny virions. To mitigate the inextricable dependence on host cell protein synthesis, viruses can modulate protein synthesis through a variety of mechanisms. We report that the viral protein kinase (vPK) encoded by open reading frame 36 (ORF36) of Kaposi's sarcoma-associated herpesvirus (KSHV) enhances protein synthesis by mimicking the function of the cellular protein S6 kinase (S6KB1). Similar to S6KB1, vPK phosphorylates the ribosomal S6 protein and up-regulates global protein synthesis. vPK also augments cellular proliferation and anchorage-independent growth. Furthermore, we report that both vPK and S6KB1 phosphorylate the enzyme 6-phosphofructo-2-kinase/fructose-2, 6-bisphosphatase 2 (PFKFB2) and that both kinases promote endothelial capillary tubule formation.
Keywords: KSHV; ORF36; S6K; cell signaling; viral protein kinase.
Conflict of interest statement
The authors declare no conflict of interest.
Figures
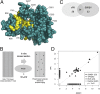
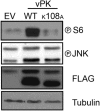
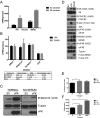
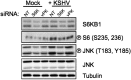
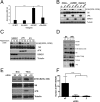
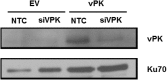
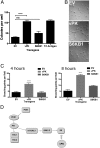
Similar articles
-
Human herpesvirus-encoded kinase induces B cell lymphomas in vivo.J Clin Invest. 2018 Jun 1;128(6):2519-2534. doi: 10.1172/JCI97053. Epub 2018 May 7. J Clin Invest. 2018. PMID: 29733294 Free PMC article.
-
Kaposi's sarcoma-associated herpesvirus viral protein kinase augments cell survival.Cell Death Dis. 2023 Oct 18;14(10):688. doi: 10.1038/s41419-023-06193-1. Cell Death Dis. 2023. PMID: 37852997 Free PMC article.
-
Kaposi's sarcoma-associated herpesvirus-encoded protein kinase and its interaction with K-bZIP.J Virol. 2007 Feb;81(3):1072-82. doi: 10.1128/JVI.01473-06. Epub 2006 Nov 15. J Virol. 2007. PMID: 17108053 Free PMC article.
-
KSHV Viral Protein Kinase Interacts with USP9X to Modulate the Viral Lifecycle.J Virol. 2023 Mar 30;97(3):e0176322. doi: 10.1128/jvi.01763-22. Epub 2023 Mar 6. J Virol. 2023. PMID: 36995092 Free PMC article.
-
Kaposi's sarcoma-associated herpesvirus viral protein kinase phosphorylates extracellular signal-regulated kinase and activates MAPK/ERK signaling pathway.Biochem Biophys Res Commun. 2020 Jan 22;521(4):1083-1088. doi: 10.1016/j.bbrc.2019.11.038. Epub 2019 Nov 13. Biochem Biophys Res Commun. 2020. PMID: 31733836
Cited by
-
Cancers associated with human gammaherpesviruses.FEBS J. 2022 Dec;289(24):7631-7669. doi: 10.1111/febs.16206. Epub 2021 Oct 2. FEBS J. 2022. PMID: 34536980 Free PMC article. Review.
-
Lysosome Membrane Permeabilization and Disruption of the Molecular Target of Rapamycin (mTOR)-Lysosome Interaction Are Associated with the Inhibition of Lung Cancer Cell Proliferation by a Chloroquinoline Analog.Mol Pharmacol. 2019 Jan;95(1):127-138. doi: 10.1124/mol.118.113118. Epub 2018 Nov 8. Mol Pharmacol. 2019. PMID: 30409790 Free PMC article.
-
Human herpesvirus-encoded kinase induces B cell lymphomas in vivo.J Clin Invest. 2018 Jun 1;128(6):2519-2534. doi: 10.1172/JCI97053. Epub 2018 May 7. J Clin Invest. 2018. PMID: 29733294 Free PMC article.
-
Kaposi's sarcoma-associated herpesvirus at 27.Tumour Virus Res. 2021 Dec;12:200223. doi: 10.1016/j.tvr.2021.200223. Epub 2021 Jun 19. Tumour Virus Res. 2021. PMID: 34153523 Free PMC article. Review.
-
Kaposi sarcoma-associated herpesvirus miRNAs suppress CASTOR1-mediated mTORC1 inhibition to promote tumorigenesis.J Clin Invest. 2019 Jul 15;129(8):3310-3323. doi: 10.1172/JCI127166. eCollection 2019 Jul 15. J Clin Invest. 2019. PMID: 31305263 Free PMC article.
References
-
- Manning G, Whyte DB, Martinez R, Hunter T, Sudarsanam S. The protein kinase complement of the human genome. Science. 2002;298(5600):1912–1934. - PubMed
-
- Ma XM, Blenis J. Molecular mechanisms of mTOR-mediated translational control. Nat Rev Mol Cell Biol. 2009;10(5):307–318. - PubMed
-
- Fenton TR, Gout IT. Functions and regulation of the 70kDa ribosomal S6 kinases. Int J Biochem Cell Biol. 2011;43(1):47–59. - PubMed
Publication types
MeSH terms
Substances
Grants and funding
LinkOut - more resources
Full Text Sources
Other Literature Sources
Research Materials