Trafficking of Endogenous Immunoglobulins by Endothelial Cells at the Blood-Brain Barrier
- PMID: 27149947
- PMCID: PMC4858719
- DOI: 10.1038/srep25658
Trafficking of Endogenous Immunoglobulins by Endothelial Cells at the Blood-Brain Barrier
Abstract
The Blood-Brain Barrier (BBB) restricts access of large molecules to the brain. The low endocytic activity of brain endothelial cells (BECs) is believed to limit delivery of immunoglobulins (IgG) to the brain parenchyma. Here, we report that endogenous mouse IgG are localized within intracellular vesicles at steady state in BECs in vivo. Using high-resolution quantitative microscopy, we found a fraction of endocytosed IgG in lysosomes. We observed that loss of pericytes (key components of the BBB) in pdgf-b(ret/ret) mice affects the intracellular distribution of endogenous mouse IgG in BECs. In these mice, endogenous IgG was not detected within lysosomes but instead accumulate at the basement membrane and brain parenchyma. Such IgG accumulation could be due to reduced lysosomal clearance and increased sorting to the abluminal membrane of BECs. Our results suggest that, in addition to low uptake from circulation, IgG lysosomal degradation may be a downstream mechanism by which BECs further restrict IgG access to the brain.
Conflict of interest statement
R.V., L.O., F.G., H.L., P.-O.F. and L.C. are all are under paid employment by the company F. Hoffmann-La Roche. R.V. is a Roche post-doctoral fellow.
Figures
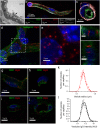
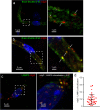
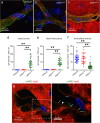
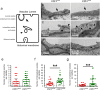
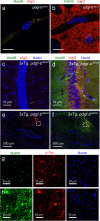
Similar articles
-
Region-specific permeability of the blood-brain barrier upon pericyte loss.J Cereb Blood Flow Metab. 2017 Dec;37(12):3683-3694. doi: 10.1177/0271678X17697340. Epub 2017 Mar 1. J Cereb Blood Flow Metab. 2017. PMID: 28273726 Free PMC article.
-
A new blood-brain barrier model using primary rat brain endothelial cells, pericytes and astrocytes.Neurochem Int. 2009 Mar-Apr;54(3-4):253-63. doi: 10.1016/j.neuint.2008.12.002. Epub 2008 Dec 7. Neurochem Int. 2009. PMID: 19111869
-
Sorting Tubules Regulate Blood-Brain Barrier Transcytosis.Cell Rep. 2017 Dec 12;21(11):3256-3270. doi: 10.1016/j.celrep.2017.11.055. Cell Rep. 2017. PMID: 29241551
-
Blood-brain barrier delivery for lysosomal storage disorders with IgG-lysosomal enzyme fusion proteins.Adv Drug Deliv Rev. 2022 May;184:114234. doi: 10.1016/j.addr.2022.114234. Epub 2022 Mar 17. Adv Drug Deliv Rev. 2022. PMID: 35307484 Review.
-
The abluminal endothelial membrane in neurovascular remodeling in health and disease.Sci Signal. 2012 Aug 7;5(236):re4. doi: 10.1126/scisignal.2002886. Sci Signal. 2012. PMID: 22871611 Review.
Cited by
-
Advances in Intrathecal Nanoparticle Delivery: Targeting the Blood-Cerebrospinal Fluid Barrier for Enhanced CNS Drug Delivery.Pharmaceuticals (Basel). 2024 Aug 15;17(8):1070. doi: 10.3390/ph17081070. Pharmaceuticals (Basel). 2024. PMID: 39204177 Free PMC article. Review.
-
Blood-based therapies to combat neurodegenerative diseases.Metab Brain Dis. 2024 Jun;39(5):985-1004. doi: 10.1007/s11011-024-01368-x. Epub 2024 Jun 6. Metab Brain Dis. 2024. PMID: 38842660 Review.
-
Strategies to identify, engineer, and validate antibodies targeting blood-brain barrier receptor-mediated transcytosis systems for CNS drug delivery.Expert Opin Drug Deliv. 2023 Jul-Dec;20(12):1789-1800. doi: 10.1080/17425247.2023.2286371. Epub 2023 Dec 29. Expert Opin Drug Deliv. 2023. PMID: 38007619 Review.
-
Delivery of the Brainshuttle™ amyloid-beta antibody fusion trontinemab to non-human primate brain and projected efficacious dose regimens in humans.MAbs. 2023 Jan-Dec;15(1):2261509. doi: 10.1080/19420862.2023.2261509. Epub 2023 Oct 12. MAbs. 2023. PMID: 37823690 Free PMC article.
-
Nano-immunotherapy: overcoming delivery challenge of immune checkpoint therapy.J Nanobiotechnology. 2023 Sep 21;21(1):339. doi: 10.1186/s12951-023-02083-y. J Nanobiotechnology. 2023. PMID: 37735656 Free PMC article. Review.
References
Publication types
MeSH terms
Substances
Grants and funding
LinkOut - more resources
Full Text Sources
Other Literature Sources