Microencapsulated equine mesenchymal stromal cells promote cutaneous wound healing in vitro
- PMID: 25889766
- PMCID: PMC4413990
- DOI: 10.1186/s13287-015-0037-x
Microencapsulated equine mesenchymal stromal cells promote cutaneous wound healing in vitro
Abstract
Introduction: The prevalence of impaired cutaneous wound healing is high and treatment is difficult and often ineffective, leading to negative social and economic impacts for our society. Innovative treatments to improve cutaneous wound healing by promoting complete tissue regeneration are therefore urgently needed. Mesenchymal stromal cells (MSCs) have been reported to provide paracrine signals that promote wound healing, but (i) how they exert their effects on target cells is unclear and (ii) a suitable delivery system to supply these MSC-derived secreted factors in a controlled and safe way is unavailable. The present study was designed to provide answers to these questions by using the horse as a translational model. Specifically, we aimed to (i) evaluate the in vitro effects of equine MSC-derived conditioned medium (CM), containing all factors secreted by MSCs, on equine dermal fibroblasts, a cell type critical for successful wound healing, and (ii) explore the potential of microencapsulated equine MSCs to deliver CM to wounded cells in vitro.
Methods: MSCs were isolated from the peripheral blood of healthy horses. Equine dermal fibroblasts from the NBL-6 (horse dermal fibroblast cell) line were wounded in vitro, and cell migration and expression levels of genes involved in wound healing were evaluated after treatment with MSC-CM or NBL-6-CM. These assays were repeated by using the CM collected from MSCs encapsulated in core-shell hydrogel microcapsules.
Results: Our salient findings were that equine MSC-derived CM stimulated the migration of equine dermal fibroblasts and increased their expression level of genes that positively contribute to wound healing. In addition, we found that equine MSCs packaged in core-shell hydrogel microcapsules had similar effects on equine dermal fibroblast migration and gene expression, indicating that microencapsulation of MSCs does not interfere with the release of bioactive factors.
Conclusions: Our results demonstrate that the use of CM from MSCs might be a promising new therapy for impaired cutaneous wounds and that encapsulation may be a suitable way to effectively deliver CM to wounded cells in vivo.
Figures
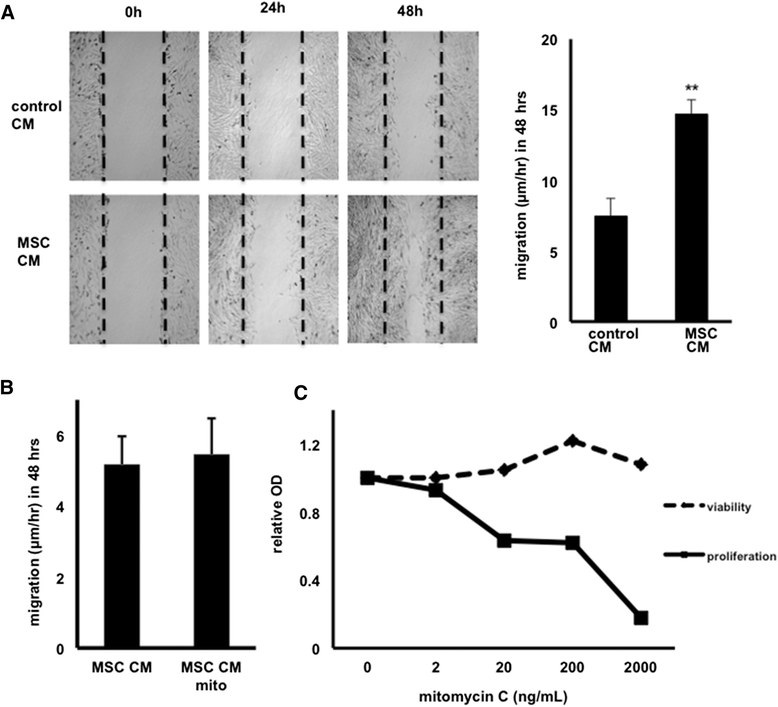
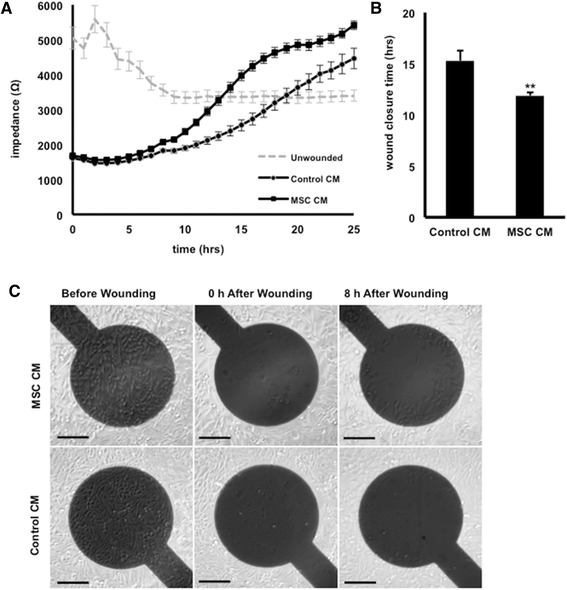
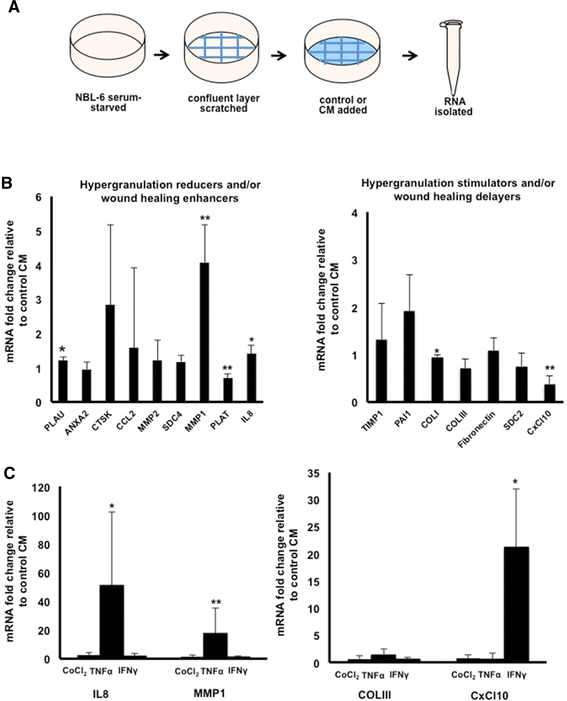
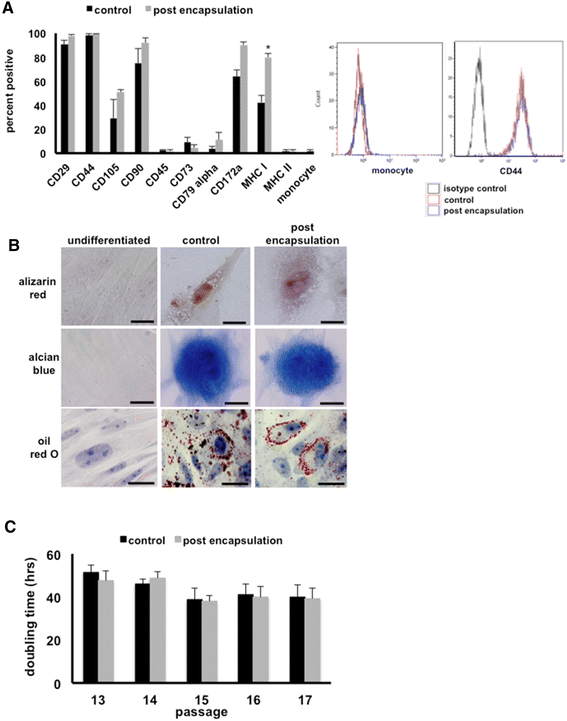
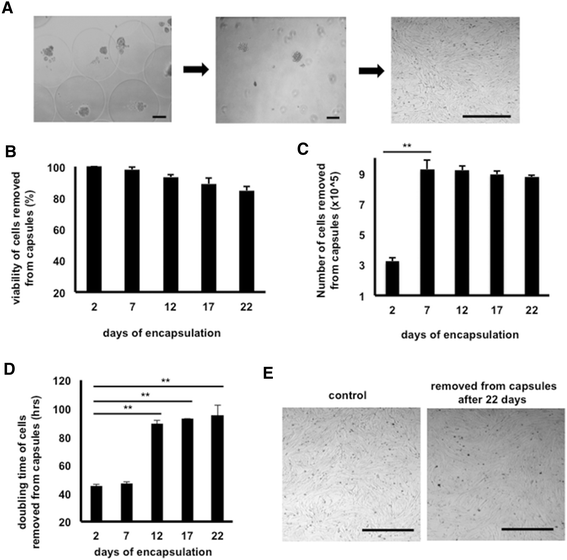
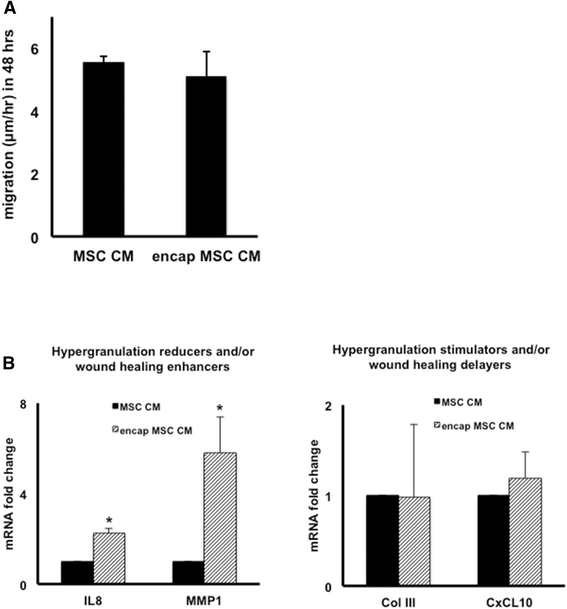
Similar articles
-
Plasminogen activator inhibitor-1 and tenascin-C secreted by equine mesenchymal stromal cells stimulate dermal fibroblast migration in vitro and contribute to wound healing in vivo.Cytotherapy. 2018 Aug;20(8):1061-1076. doi: 10.1016/j.jcyt.2018.06.005. Epub 2018 Aug 4. Cytotherapy. 2018. PMID: 30087008
-
Secreted factors from equine mesenchymal stromal cells diminish the effects of TGF-β1 on equine dermal fibroblasts and alter the phenotype of dermal fibroblasts isolated from cutaneous fibroproliferative wounds.Wound Repair Regen. 2017 Apr;25(2):234-247. doi: 10.1111/wrr.12515. Epub 2017 Apr 27. Wound Repair Regen. 2017. PMID: 28370679
-
Mesenchymal stem cell-conditioned medium accelerates wound healing with fewer scars.Int Wound J. 2017 Feb;14(1):64-73. doi: 10.1111/iwj.12551. Epub 2015 Dec 3. Int Wound J. 2017. PMID: 26635066 Free PMC article.
-
In vitro study of the mesenchymal stem cells-conditional media role in skin wound healing process: A systematic review.Int Wound J. 2022 Dec;19(8):2210-2223. doi: 10.1111/iwj.13796. Epub 2022 Apr 12. Int Wound J. 2022. PMID: 35412017 Free PMC article. Review.
-
Role of Mesenchymal Stem Cells in Dermal Repair in Burns and Diabetic Wounds.Curr Stem Cell Res Ther. 2017;12(1):61-70. doi: 10.2174/1574888x11666160714115926. Curr Stem Cell Res Ther. 2017. PMID: 27412677 Review.
Cited by
-
The Lack of a Representative Tendinopathy Model Hampers Fundamental Mesenchymal Stem Cell Research.Front Cell Dev Biol. 2021 May 3;9:651164. doi: 10.3389/fcell.2021.651164. eCollection 2021. Front Cell Dev Biol. 2021. PMID: 34012963 Free PMC article. Review.
-
Direct Differentiation of Human Embryonic Stem Cells to 3D Functional Hepatocyte-like Cells in Alginate Microencapsulation Sphere.Cells. 2022 Oct 5;11(19):3134. doi: 10.3390/cells11193134. Cells. 2022. PMID: 36231094 Free PMC article.
-
Electric Cell-Substrate Impedance Sensing To Monitor Viral Growth and Study Cellular Responses to Infection with Alphaherpesviruses in Real Time.mSphere. 2017 Apr 5;2(2):e00039-17. doi: 10.1128/mSphere.00039-17. eCollection 2017 Mar-Apr. mSphere. 2017. PMID: 28405631 Free PMC article.
-
Antimicrobial peptides secreted by equine mesenchymal stromal cells inhibit the growth of bacteria commonly found in skin wounds.Stem Cell Res Ther. 2017 Jul 4;8(1):157. doi: 10.1186/s13287-017-0610-6. Stem Cell Res Ther. 2017. PMID: 28676123 Free PMC article.
-
Effects of Botulinum Toxin Type A on Microvessels in Hypertrophic Scar Models on Rabbit Ears.Biomed Res Int. 2020 Jun 16;2020:2170750. doi: 10.1155/2020/2170750. eCollection 2020. Biomed Res Int. 2020. PMID: 32626735 Free PMC article.
References
Publication types
MeSH terms
Substances
LinkOut - more resources
Full Text Sources
Other Literature Sources