Transient receptor potential channels in the vasculature
- PMID: 25834234
- PMCID: PMC4551213
- DOI: 10.1152/physrev.00026.2014
Transient receptor potential channels in the vasculature
Abstract
The mammalian genome encodes 28 distinct members of the transient receptor potential (TRP) superfamily of cation channels, which exhibit varying degrees of selectivity for different ionic species. Multiple TRP channels are present in all cells and are involved in diverse aspects of cellular function, including sensory perception and signal transduction. Notably, TRP channels are involved in regulating vascular function and pathophysiology, the focus of this review. TRP channels in vascular smooth muscle cells participate in regulating contractility and proliferation, whereas endothelial TRP channel activity is an important contributor to endothelium-dependent vasodilation, vascular wall permeability, and angiogenesis. TRP channels are also present in perivascular sensory neurons and astrocytic endfeet proximal to cerebral arterioles, where they participate in the regulation of vascular tone. Almost all of these functions are mediated by changes in global intracellular Ca(2+) levels or subcellular Ca(2+) signaling events. In addition to directly mediating Ca(2+) entry, TRP channels influence intracellular Ca(2+) dynamics through membrane depolarization associated with the influx of cations or through receptor- or store-operated mechanisms. Dysregulation of TRP channels is associated with vascular-related pathologies, including hypertension, neointimal injury, ischemia-reperfusion injury, pulmonary edema, and neurogenic inflammation. In this review, we briefly consider general aspects of TRP channel biology and provide an in-depth discussion of the functions of TRP channels in vascular smooth muscle cells, endothelial cells, and perivascular cells under normal and pathophysiological conditions.
Copyright © 2015 the American Physiological Society.
Figures
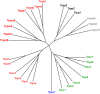
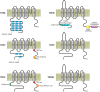
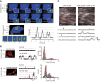
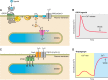
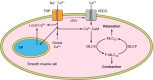
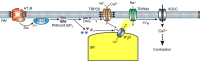
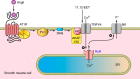
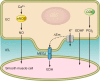
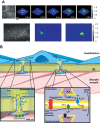
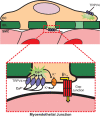
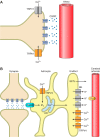
Similar articles
-
Endothelium-dependent cerebral artery dilation mediated by transient receptor potential and Ca2+-activated K+ channels.J Cardiovasc Pharmacol. 2011 Feb;57(2):148-53. doi: 10.1097/FJC.0b013e3181f580d9. J Cardiovasc Pharmacol. 2011. PMID: 20729757 Review.
-
TRP channels and the control of vascular function.Curr Opin Pharmacol. 2010 Apr;10(2):127-32. doi: 10.1016/j.coph.2009.11.010. Epub 2010 Jan 7. Curr Opin Pharmacol. 2010. PMID: 20060363 Review.
-
Muscling in on TRP channels in vascular smooth muscle cells and cardiomyocytes.Cell Calcium. 2017 Sep;66:48-61. doi: 10.1016/j.ceca.2017.06.004. Epub 2017 Jun 15. Cell Calcium. 2017. PMID: 28807149 Review.
-
Pathophysiological implications of transient receptor potential channels in vascular function.Curr Opin Nephrol Hypertens. 2008 Mar;17(2):193-8. doi: 10.1097/MNH.0b013e3282f52467. Curr Opin Nephrol Hypertens. 2008. PMID: 18277154 Review.
-
Role of transient receptor potential channels in the regulation of vascular tone.Drug Discov Today. 2024 Jul;29(7):104051. doi: 10.1016/j.drudis.2024.104051. Epub 2024 Jun 3. Drug Discov Today. 2024. PMID: 38838960 Review.
Cited by
-
Zinc drives vasorelaxation by acting in sensory nerves, endothelium and smooth muscle.Nat Commun. 2021 Jun 1;12(1):3296. doi: 10.1038/s41467-021-23198-6. Nat Commun. 2021. PMID: 34075043 Free PMC article.
-
Sevoflurane preconditioning promotes mesenchymal stem cells to relieve myocardial ischemia/reperfusion injury via TRPC6-induced angiogenesis.Stem Cell Res Ther. 2021 Nov 22;12(1):584. doi: 10.1186/s13287-021-02649-3. Stem Cell Res Ther. 2021. PMID: 34809715 Free PMC article.
-
Potential of the TRPM7 channel as a novel therapeutic target for pulmonary arterial hypertension.J Smooth Muscle Res. 2022;58(0):50-62. doi: 10.1540/jsmr.58.50. J Smooth Muscle Res. 2022. PMID: 35944979 Free PMC article. Review.
-
TRPV4 channels contribute to renal myogenic autoregulation in neonatal pigs.Am J Physiol Renal Physiol. 2017 Nov 1;313(5):F1136-F1148. doi: 10.1152/ajprenal.00300.2017. Epub 2017 Aug 2. Am J Physiol Renal Physiol. 2017. PMID: 28768667 Free PMC article.
-
Crosslink between calcium and sodium signalling.Exp Physiol. 2018 Feb 1;103(2):157-169. doi: 10.1113/EP086534. Epub 2018 Jan 16. Exp Physiol. 2018. PMID: 29210126 Free PMC article. Review.
References
-
- Aarts M, Iihara K, Wei WL, Xiong ZG, Arundine M, Cerwinski W, MacDonald JF, Tymianski M. A key role for TRPM7 channels in anoxic neuronal death. Cell 115: 863–877, 2003. - PubMed
-
- Adebiyi A, Thomas-Gatewood CM, Leo MD, Kidd MW, Neeb ZP, Jaggar JH. An elevation in physical coupling of type 1 inositol 1,4,5-trisphosphate (IP3) receptors to transient receptor potential 3 (TRPC3) channels constricts mesenteric arteries in genetic hypertension. Hypertension 60: 1213–1219, 2012. - PMC - PubMed
Publication types
MeSH terms
Substances
Grants and funding
LinkOut - more resources
Full Text Sources
Other Literature Sources
Miscellaneous