Irradiation and anti-PD-L1 treatment synergistically promote antitumor immunity in mice
- PMID: 24382348
- PMCID: PMC3904601
- DOI: 10.1172/JCI67313
Irradiation and anti-PD-L1 treatment synergistically promote antitumor immunity in mice
Abstract
High-dose ionizing irradiation (IR) results in direct tumor cell death and augments tumor-specific immunity, which enhances tumor control both locally and distantly. Unfortunately, local relapses often occur following IR treatment, indicating that IR-induced responses are inadequate to maintain antitumor immunity. Therapeutic blockade of the T cell negative regulator programmed death-ligand 1 (PD-L1, also called B7-H1) can enhance T cell effector function when PD-L1 is expressed in chronically inflamed tissues and tumors. Here, we demonstrate that PD-L1 was upregulated in the tumor microenvironment after IR. Administration of anti-PD-L1 enhanced the efficacy of IR through a cytotoxic T cell-dependent mechanism. Concomitant with IR-mediated tumor regression, we observed that IR and anti-PD-L1 synergistically reduced the local accumulation of tumor-infiltrating myeloid-derived suppressor cells (MDSCs), which suppress T cells and alter the tumor immune microenvironment. Furthermore, activation of cytotoxic T cells with combination therapy mediated the reduction of MDSCs in tumors through the cytotoxic actions of TNF. Our data provide evidence for a close interaction between IR, T cells, and the PD-L1/PD-1 axis and establish a basis for the rational design of combination therapy with immune modulators and radiotherapy.
Figures
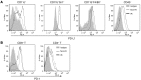
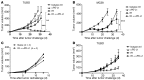
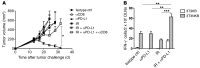
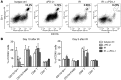
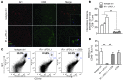
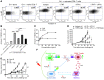
Similar articles
-
Inhibition of myeloid-derived suppressive cell function with all-trans retinoic acid enhanced anti-PD-L1 efficacy in cervical cancer.Sci Rep. 2022 Jun 10;12(1):9619. doi: 10.1038/s41598-022-13855-1. Sci Rep. 2022. PMID: 35688951 Free PMC article.
-
Combination of Sunitinib and PD-L1 Blockade Enhances Anticancer Efficacy of TLR7/8 Agonist-Based Nanovaccine.Mol Pharm. 2019 Mar 4;16(3):1200-1210. doi: 10.1021/acs.molpharmaceut.8b01165. Epub 2019 Jan 25. Mol Pharm. 2019. PMID: 30620878
-
Nanoscale Reduced Graphene Oxide-Mediated Photothermal Therapy Together with IDO Inhibition and PD-L1 Blockade Synergistically Promote Antitumor Immunity.ACS Appl Mater Interfaces. 2019 Jan 16;11(2):1876-1885. doi: 10.1021/acsami.8b18751. Epub 2019 Jan 7. ACS Appl Mater Interfaces. 2019. PMID: 30582788
-
Effect of radiotherapy on T cell and PD-1 / PD-L1 blocking therapy in tumor microenvironment.Hum Vaccin Immunother. 2021 Jun 3;17(6):1555-1567. doi: 10.1080/21645515.2020.1840254. Epub 2021 Jan 11. Hum Vaccin Immunother. 2021. PMID: 33428533 Free PMC article. Review.
-
The role of radiotherapy in tumor immunity and the potential of PET/CT in detecting the expression of PD-1/PD-L1.Jpn J Radiol. 2024 Apr;42(4):347-353. doi: 10.1007/s11604-023-01507-x. Epub 2023 Nov 13. Jpn J Radiol. 2024. PMID: 37953364 Review.
Cited by
-
Radiation meets immunotherapy - a perfect match in the era of combination therapy?Int J Radiat Biol. 2015 Apr;91(4):299-305. doi: 10.3109/09553002.2014.995383. Epub 2015 Feb 9. Int J Radiat Biol. 2015. PMID: 25630486 Free PMC article. Review.
-
The tumour microenvironment after radiotherapy: mechanisms of resistance and recurrence.Nat Rev Cancer. 2015 Jul;15(7):409-25. doi: 10.1038/nrc3958. Nat Rev Cancer. 2015. PMID: 26105538 Free PMC article. Review.
-
Radiation therapy and the abscopal effect: a concept comes of age.Ann Transl Med. 2016 Mar;4(6):118. doi: 10.21037/atm.2016.01.32. Ann Transl Med. 2016. PMID: 27127771 Free PMC article. No abstract available.
-
Safety and efficacy of radiotherapy combined with chemotherapy for recurrent metastatic renal pelvic and ureteral carcinoma.World J Urol. 2024 Jan 10;42(1):23. doi: 10.1007/s00345-023-04701-8. World J Urol. 2024. PMID: 38197979
-
ANTI-TUMOR IMMUNE RESPONSES INDUCED BY RADIOTHERAPY: A REVIEW.Fukushima J Med Sci. 2015;61(1):13-22. doi: 10.5387/fms.2015-6. Epub 2015 Jul 2. Fukushima J Med Sci. 2015. PMID: 26135666 Free PMC article. Review.
References
-
- Apetoh L, et al. Toll-like receptor 4-dependent contribution of the immune system to anticancer chemotherapy and radiotherapy. Nat Med. 2007;13(9):1050–1059. - PubMed
-
- Barcellos-Hoff MH, Park C, Wright EG. Radiation and the microenvironment - tumorigenesis and therapy. Nat Rev Cancer. 2005;5(11):867–875. - PubMed
-
- Fridman WH, Pages F, Sautes-Fridman C, Galon J. The immune contexture in human tumours: impact on clinical outcome. Nat Rev Cancer. 2012;12(4):298–306. - PubMed
Publication types
MeSH terms
Substances
Grants and funding
LinkOut - more resources
Full Text Sources
Other Literature Sources
Molecular Biology Databases
Research Materials