A structural basis for BRD2/4-mediated host chromatin interaction and oligomer assembly of Kaposi sarcoma-associated herpesvirus and murine gammaherpesvirus LANA proteins
- PMID: 24146614
- PMCID: PMC3798688
- DOI: 10.1371/journal.ppat.1003640
A structural basis for BRD2/4-mediated host chromatin interaction and oligomer assembly of Kaposi sarcoma-associated herpesvirus and murine gammaherpesvirus LANA proteins
Abstract
Kaposi sarcoma-associated herpesvirus (KSHV) establishes a lifelong latent infection and causes several malignancies in humans. Murine herpesvirus 68 (MHV-68) is a related γ2-herpesvirus frequently used as a model to study the biology of γ-herpesviruses in vivo. The KSHV latency-associated nuclear antigen (kLANA) and the MHV68 mLANA (orf73) protein are required for latent viral replication and persistence. Latent episomal KSHV genomes and kLANA form nuclear microdomains, termed 'LANA speckles', which also contain cellular chromatin proteins, including BRD2 and BRD4, members of the BRD/BET family of chromatin modulators. We solved the X-ray crystal structure of the C-terminal DNA binding domains (CTD) of kLANA and MHV-68 mLANA. While these structures share the overall fold with the EBNA1 protein of Epstein-Barr virus, they differ substantially in their surface characteristics. Opposite to the DNA binding site, both kLANA and mLANA CTD contain a characteristic lysine-rich positively charged surface patch, which appears to be a unique feature of γ2-herpesviral LANA proteins. Importantly, kLANA and mLANA CTD dimers undergo higher order oligomerization. Using NMR spectroscopy we identified a specific binding site for the ET domains of BRD2/4 on kLANA. Functional studies employing multiple kLANA mutants indicate that the oligomerization of native kLANA CTD dimers, the characteristic basic patch and the ET binding site on the kLANA surface are required for the formation of kLANA 'nuclear speckles' and latent replication. Similarly, the basic patch on mLANA contributes to the establishment of MHV-68 latency in spleen cells in vivo. In summary, our data provide a structural basis for the formation of higher order LANA oligomers, which is required for nuclear speckle formation, latent replication and viral persistence.
Conflict of interest statement
The authors have declared that no competing interests exist.
Figures
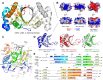
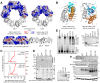
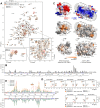
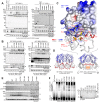
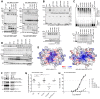
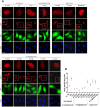
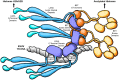
Similar articles
-
Murine Gammaherpesvirus 68 Expressing Kaposi Sarcoma-Associated Herpesvirus Latency-Associated Nuclear Antigen (LANA) Reveals both Functional Conservation and Divergence in LANA Homologs.J Virol. 2017 Sep 12;91(19):e00992-17. doi: 10.1128/JVI.00992-17. Print 2017 Oct 1. J Virol. 2017. PMID: 28747501 Free PMC article.
-
In Vivo Persistence of Chimeric Virus after Substitution of the Kaposi's Sarcoma-Associated Herpesvirus LANA DNA Binding Domain with That of Murid Herpesvirus 4.J Virol. 2018 Oct 12;92(21):e01251-18. doi: 10.1128/JVI.01251-18. Print 2018 Nov 1. J Virol. 2018. PMID: 30111565 Free PMC article.
-
Brd/BET Proteins Influence the Genome-Wide Localization of the Kaposi's Sarcoma-Associated Herpesvirus and Murine Gammaherpesvirus Major Latency Proteins.Front Microbiol. 2020 Oct 22;11:591778. doi: 10.3389/fmicb.2020.591778. eCollection 2020. Front Microbiol. 2020. PMID: 33193257 Free PMC article.
-
Kaposi's Sarcoma-Associated Herpesvirus Latency-Associated Nuclear Antigen: Replicating and Shielding Viral DNA during Viral Persistence.J Virol. 2017 Jun 26;91(14):e01083-16. doi: 10.1128/JVI.01083-16. Print 2017 Jul 15. J Virol. 2017. PMID: 28446671 Free PMC article. Review.
-
KSHV LANA--the master regulator of KSHV latency.Viruses. 2014 Dec 11;6(12):4961-98. doi: 10.3390/v6124961. Viruses. 2014. PMID: 25514370 Free PMC article. Review.
Cited by
-
Disrupting Kaposi's Sarcoma-Associated Herpesvirus (KSHV) Latent Replication with a Small Molecule Inhibitor.J Med Chem. 2023 Aug 10;66(15):10782-10790. doi: 10.1021/acs.jmedchem.3c00990. Epub 2023 Jul 28. J Med Chem. 2023. PMID: 37506283 Free PMC article.
-
Chicken bromodomain-containing protein 2 interacts with the Newcastle disease virus matrix protein and promotes viral replication.Vet Res. 2020 Sep 22;51(1):120. doi: 10.1186/s13567-020-00846-1. Vet Res. 2020. PMID: 32962745 Free PMC article.
-
Enhancer-promoter activation by the Kaposi sarcoma-associated herpesvirus episome maintenance protein LANA.Cell Rep. 2024 Mar 26;43(3):113888. doi: 10.1016/j.celrep.2024.113888. Epub 2024 Feb 27. Cell Rep. 2024. PMID: 38416644 Free PMC article.
-
The Enigmatic Origin of Papillomavirus Protein Domains.Viruses. 2017 Aug 23;9(9):240. doi: 10.3390/v9090240. Viruses. 2017. PMID: 28832519 Free PMC article.
-
Kaposi's sarcoma-associated herpesvirus (KSHV) LANA prevents KSHV episomes from degradation.J Virol. 2024 Feb 20;98(2):e0126823. doi: 10.1128/jvi.01268-23. Epub 2024 Jan 19. J Virol. 2024. PMID: 38240588 Free PMC article.
References
-
- Schulz TF (2006) The pleiotropic effects of Kaposi's sarcoma herpesvirus. J Pathol 208: 187–198. - PubMed
-
- Rainbow L, Platt GM, Simpson GR, Sarid R, Gao SJ, et al. (1997) The 222- to 234-kilodalton latent nuclear protein (LNA) of Kaposi's sarcoma-associated herpesvirus (human herpesvirus 8) is encoded by orf73 and is a component of the latency-associated nuclear antigen. J Virol 71: 5915–5921. - PMC - PubMed
-
- Garber AC, Hu J, Renne R (2002) Latency-associated nuclear antigen (LANA) cooperatively binds to two sites within the terminal repeat, and both sites contribute to the ability of LANA to suppress transcription and to facilitate DNA replication. JBiolChem 277: 27401–27411. - PubMed
Publication types
MeSH terms
Substances
Associated data
- Actions
- Actions
- Actions
- Actions
Grants and funding
LinkOut - more resources
Full Text Sources
Other Literature Sources
Molecular Biology Databases
Miscellaneous