NF-κB RelB negatively regulates osteoblast differentiation and bone formation
- PMID: 24115294
- PMCID: PMC3961566
- DOI: 10.1002/jbmr.2108
NF-κB RelB negatively regulates osteoblast differentiation and bone formation
Abstract
RelA-mediated NF-κB canonical signaling promotes mesenchymal progenitor cell (MPC) proliferation, but inhibits differentiation of mature osteoblasts (OBs) and thus negatively regulates bone formation. Previous studies suggest that NF-κB RelB may also negatively regulate bone formation through noncanonical signaling, but they involved a complex knockout mouse model, and the molecular mechanisms involved were not investigated. Here, we report that RelB(-/-) mice develop age-related increased trabecular bone mass associated with increased bone formation. RelB(-/-) bone marrow stromal cells expanded faster in vitro and have enhanced OB differentiation associated with increased expression of the osteoblastogenic transcription factor, Runt-related transcription factor 2 (Runx2). In addition, RelB directly targeted the Runx2 promoter to inhibit its activation. Importantly, RelB(-/-) bone-derived MPCs formed bone more rapidly than wild-type cells after they were injected into a murine tibial bone defect model. Our findings indicate that RelB negatively regulates bone mass as mice age and limits bone formation in healing bone defects, suggesting that inhibition of RelB could reduce age-related bone loss and enhance bone repair.
Keywords: BONE FORMATION; MESENCHYMAL PROGENITOR CELLS; NF-κB; OSTEOBLASTS; RELB.
© 2014 American Society for Bone and Mineral Research.
Conflict of interest statement
The authors have declared that no conflict of interest exists.
Figures
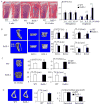
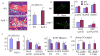
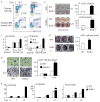
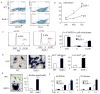
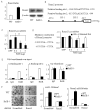
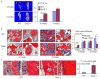
Similar articles
-
NOTCH inhibits osteoblast formation in inflammatory arthritis via noncanonical NF-κB.J Clin Invest. 2014 Jul;124(7):3200-14. doi: 10.1172/JCI68901. Epub 2014 Jun 2. J Clin Invest. 2014. PMID: 24892805 Free PMC article.
-
Noncanonical NF-κB signaling regulates hematopoietic stem cell self-renewal and microenvironment interactions.Stem Cells. 2012 Apr;30(4):709-18. doi: 10.1002/stem.1050. Stem Cells. 2012. PMID: 22290873 Free PMC article.
-
Ubiquitin e3 ligase itch negatively regulates osteoblast differentiation from mesenchymal progenitor cells.Stem Cells. 2013 Aug;31(8):1574-83. doi: 10.1002/stem.1395. Stem Cells. 2013. PMID: 23606569 Free PMC article.
-
RelB: an outlier in leukocyte biology.J Leukoc Biol. 2013 Nov;94(5):941-51. doi: 10.1189/jlb.0513305. Epub 2013 Aug 6. J Leukoc Biol. 2013. PMID: 23922380 Free PMC article. Review.
-
[NF-κB signaling pathways and the future perspectives of bone disease therapy using selective inhibitors of NF-κB].Clin Calcium. 2016 Feb;26(2):298-304. Clin Calcium. 2016. PMID: 26813510 Review. Japanese.
Cited by
-
Protective effects of resveratrol on osteoporosis via activation of the SIRT1-NF-κB signaling pathway in rats.Exp Ther Med. 2017 Nov;14(5):5032-5038. doi: 10.3892/etm.2017.5147. Epub 2017 Sep 20. Exp Ther Med. 2017. PMID: 29201210 Free PMC article.
-
The IRF2BP2-KLF2 axis regulates osteoclast and osteoblast differentiation.BMB Rep. 2019 Jul;52(7):469-474. doi: 10.5483/BMBRep.2019.52.7.104. BMB Rep. 2019. PMID: 31186082 Free PMC article.
-
Role and mechanism of action of leucine-rich repeat kinase 1 in bone.Bone Res. 2017 Mar 14;5:17003. doi: 10.1038/boneres.2017.3. eCollection 2017. Bone Res. 2017. PMID: 28326224 Free PMC article. Review.
-
Manipulation of the Alternative NF-κB Pathway in Mice Has Sexually Dimorphic Effects on Bone.JBMR Plus. 2018 Aug 23;3(1):14-22. doi: 10.1002/jbm4.10066. eCollection 2019 Jan. JBMR Plus. 2018. PMID: 30680359 Free PMC article.
-
Critical Roles of NF-κB Signaling Molecules in Bone Metabolism Revealed by Genetic Mutations in Osteopetrosis.Int J Mol Sci. 2022 Jul 20;23(14):7995. doi: 10.3390/ijms23147995. Int J Mol Sci. 2022. PMID: 35887342 Free PMC article. Review.
References
-
- Mundy GR. Osteoporosis and inflammation. Nutr Rev. 2007;65(12 Pt 2):S147–51. - PubMed
-
- Vallabhapurapu S, Karin M. Regulation and function of NF-kappaB transcription factors in the immune system. Annu Rev Immunol. 2009;27:693–733. - PubMed
-
- Iotsova V, Caamaño J, Loy J, Yang Y, Lewin A, Bravo R. Osteopetrosis in mice lacking NF-kappaB1 and NF-kappaB2. Nat Med. 1997;3(11):1285–9. - PubMed
-
- Yamashita T, Yao Z, Li F, et al. NF-kappaB p50 and p52 regulate receptor activator of NF-kappaB ligand (RANKL) and tumor necrosis factor-induced osteoclast precursor differentiation by activating c-Fos and NFATc1. J Biol Chem. 2007;282(25):18245–53. - PubMed
Publication types
MeSH terms
Substances
Grants and funding
LinkOut - more resources
Full Text Sources
Other Literature Sources
Molecular Biology Databases
Miscellaneous