The redox proteome
- PMID: 23861437
- PMCID: PMC3772199
- DOI: 10.1074/jbc.R113.464131
The redox proteome
Abstract
The redox proteome consists of reversible and irreversible covalent modifications that link redox metabolism to biologic structure and function. These modifications, especially of Cys, function at the molecular level in protein folding and maturation, catalytic activity, signaling, and macromolecular interactions and at the macroscopic level in control of secretion and cell shape. Interaction of the redox proteome with redox-active chemicals is central to macromolecular structure, regulation, and signaling during the life cycle and has a central role in the tolerance and adaptability to diet and environmental challenges.
Keywords: Glutathione; Redox Regulation; Systems Biology; Thiol; Thioredoxin.
Figures
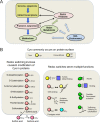
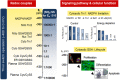
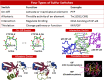
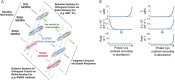
Similar articles
-
Characterization of surface-exposed reactive cysteine residues in Saccharomyces cerevisiae.Biochemistry. 2010 Sep 7;49(35):7709-21. doi: 10.1021/bi100677a. Biochemistry. 2010. PMID: 20698499 Free PMC article.
-
Inhibition of glutathione biosynthesis alters compartmental redox status and the thiol proteome in organogenesis-stage rat conceptuses.Free Radic Biol Med. 2013 Oct;63:325-37. doi: 10.1016/j.freeradbiomed.2013.05.040. Epub 2013 Jun 2. Free Radic Biol Med. 2013. PMID: 23736079 Free PMC article.
-
Cysteine/cystine couple is a newly recognized node in the circuitry for biologic redox signaling and control.FASEB J. 2004 Aug;18(11):1246-8. doi: 10.1096/fj.03-0971fje. Epub 2004 Jun 4. FASEB J. 2004. PMID: 15180957
-
Redox proteomics: identification of oxidatively modified proteins.Proteomics. 2003 Jul;3(7):1145-53. doi: 10.1002/pmic.200300435. Proteomics. 2003. PMID: 12872215 Review.
-
The cysteine proteome.Free Radic Biol Med. 2015 Jul;84:227-245. doi: 10.1016/j.freeradbiomed.2015.03.022. Epub 2015 Apr 3. Free Radic Biol Med. 2015. PMID: 25843657 Free PMC article. Review.
Cited by
-
Regulation of the redox metabolome and thiol proteome by hydrogen sulfide.Crit Rev Biochem Mol Biol. 2021 Jun;56(3):221-235. doi: 10.1080/10409238.2021.1893641. Epub 2021 Mar 15. Crit Rev Biochem Mol Biol. 2021. PMID: 33722121 Free PMC article.
-
Hydrogen Sulfide (H2S)/Polysulfides (H2Sn) Signalling and TRPA1 Channels Modification on Sulfur Metabolism.Biomolecules. 2024 Jan 19;14(1):129. doi: 10.3390/biom14010129. Biomolecules. 2024. PMID: 38275758 Free PMC article. Review.
-
Catechol-Based Capacitor for Redox-Linked Bioelectronics.ACS Appl Electron Mater. 2019 Aug 27;1(8):1337-1347. doi: 10.1021/acsaelm.9b00272. Epub 2019 Jul 3. ACS Appl Electron Mater. 2019. PMID: 32090203 Free PMC article.
-
Magnetic resonance spectroscopy studies of substance use disorders: Current landscape and potential future directions.Pharmacol Biochem Behav. 2021 Jan;200:173090. doi: 10.1016/j.pbb.2020.173090. Epub 2020 Dec 14. Pharmacol Biochem Behav. 2021. PMID: 33333132 Free PMC article. Review.
-
Selective Sensitization of Zinc Finger Protein Oxidation by Reactive Oxygen Species through Arsenic Binding.J Biol Chem. 2015 Jul 24;290(30):18361-9. doi: 10.1074/jbc.M115.663906. Epub 2015 Jun 10. J Biol Chem. 2015. PMID: 26063799 Free PMC article.
References
-
- Keilin D. (1966) The History of Cell Respiration and Cytochrome, Cambridge University Press, Cambridge
-
- Mitchell P. (1979) Keilin's respiratory chain concept and its chemiosmotic consequences. Science 206, 1148–1159 - PubMed
-
- Mason H. S., Fowlks W. L., Peterson J. (1955) Oxygen transfer and electron transport by the phenolase complex. J. Am. Chem. Soc. 77, 2914–2915
-
- Hayaishi O., Katagiri M., Rothberg S. (1955) Mechanism of the pyrocatechase reaction. J. Am. Chem. Soc. 77, 5450–5451
Publication types
MeSH terms
Substances
Grants and funding
LinkOut - more resources
Full Text Sources
Other Literature Sources