Molecular mechanisms of RNA interference
- PMID: 23654304
- PMCID: PMC5895182
- DOI: 10.1146/annurev-biophys-083012-130404
Molecular mechanisms of RNA interference
Abstract
Small RNA molecules regulate eukaryotic gene expression during development and in response to stresses including viral infection. Specialized ribonucleases and RNA-binding proteins govern the production and action of small regulatory RNAs. After initial processing in the nucleus by Drosha, precursor microRNAs (pre-miRNAs) are transported to the cytoplasm, where Dicer cleavage generates mature microRNAs (miRNAs) and short interfering RNAs (siRNAs). These double-stranded products assemble with Argonaute proteins such that one strand is preferentially selected and used to guide sequence-specific silencing of complementary target mRNAs by endonucleolytic cleavage or translational repression. Molecular structures of Dicer and Argonaute proteins, and of RNA-bound complexes, have offered exciting insights into the mechanisms operating at the heart of RNA-silencing pathways.
Figures
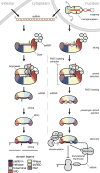
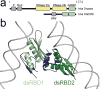
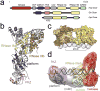
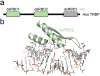
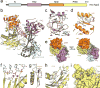
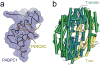
Similar articles
-
A three-dimensional view of the molecular machinery of RNA interference.Nature. 2009 Jan 22;457(7228):405-12. doi: 10.1038/nature07755. Nature. 2009. PMID: 19158786 Review.
-
Intracellular localization and routing of miRNA and RNAi pathway components.Curr Top Med Chem. 2012;12(2):79-88. doi: 10.2174/156802612798919132. Curr Top Med Chem. 2012. PMID: 22196276 Review.
-
Multiple sensors ensure guide strand selection in human RNAi pathways.RNA. 2013 May;19(5):639-48. doi: 10.1261/rna.037424.112. Epub 2013 Mar 26. RNA. 2013. PMID: 23531496 Free PMC article.
-
The microRNA Machinery.Adv Exp Med Biol. 2015;887:15-30. doi: 10.1007/978-3-319-22380-3_2. Adv Exp Med Biol. 2015. PMID: 26662984
-
Diverse small non-coding RNAs in RNA interference pathways.Methods Mol Biol. 2011;764:169-82. doi: 10.1007/978-1-61779-188-8_11. Methods Mol Biol. 2011. PMID: 21748640 Review.
Cited by
-
A SUMO E3 ligase promotes long non-coding RNA transcription to regulate small RNA-directed DNA elimination.Elife. 2024 Jan 10;13:e95337. doi: 10.7554/eLife.95337. Elife. 2024. PMID: 38197489 Free PMC article.
-
Smart functional nucleic acid chimeras: enabling tissue specific RNA targeting therapy.RNA Biol. 2015;12(4):412-25. doi: 10.1080/15476286.2015.1017234. RNA Biol. 2015. PMID: 25849197 Free PMC article. Review.
-
Non-Target Effects of dsRNA Molecules in Hemipteran Insects.Genes (Basel). 2021 Mar 12;12(3):407. doi: 10.3390/genes12030407. Genes (Basel). 2021. PMID: 33809132 Free PMC article.
-
Targeted Therapy in Cardiovascular Disease: A Precision Therapy Era.Front Pharmacol. 2021 Apr 16;12:623674. doi: 10.3389/fphar.2021.623674. eCollection 2021. Front Pharmacol. 2021. PMID: 33935716 Free PMC article. Review.
-
Small RNAs in plants: recent development and application for crop improvement.Front Plant Sci. 2015 Apr 2;6:208. doi: 10.3389/fpls.2015.00208. eCollection 2015. Front Plant Sci. 2015. PMID: 25883599 Free PMC article. Review.
References
-
- Bellemer C, Bortolin-Cavaillé M-L, Schmidt U, Jensen SMR, Kjems J, Bertrand E, Cavaillé J. Microprocessor dynamics and interactions at endogenous imprinted C19MC microRNA genes. J. Cell. Sci. 2012;125(Pt 11):2709–2720. - PubMed
-
- Berezikov E. Nat. Rev. Genet. 12. Vol. 12. Nature Publishing Group; 2011. Evolution of microRNA diversity and regulation in animals; pp. 846–860. - PubMed
-
- Bernstein E, Caudy AA, Hammond SM, Hannon GJ. Role for a bidentate ribonuclease in the initiation step of RNA interference. Nature. 2001;409(6818):363–366. - PubMed
Publication types
MeSH terms
Substances
Grants and funding
LinkOut - more resources
Full Text Sources
Other Literature Sources