An alternative Kaposi's sarcoma-associated herpesvirus replication program triggered by host cell apoptosis
- PMID: 22345480
- PMCID: PMC3318647
- DOI: 10.1128/JVI.06617-11
An alternative Kaposi's sarcoma-associated herpesvirus replication program triggered by host cell apoptosis
Abstract
Kaposi's sarcoma-associated herpesvirus (KSHV) is linked to several neoplastic diseases: Kaposi's sarcoma, primary effusion lymphoma (PEL), and multicentric Castleman's disease (MCD). KSHV replicates actively, via a controlled gene expression program, but can also remain latent. It had been thought that the transition from latent to lytic replication was controlled exclusively by the replication and transcription activator protein RTA (open reading frame 50 [ORF50] gene product). A dominant-negative (DN) ORF50 mutant, ORF50ΔSTAD, blocks gene expression and replication. We produced a PEL cell line derivative containing both latent KSHV genomes and an inducible ORF50ΔSTAD. We unexpectedly found that induction of apoptosis triggered high-level viral replication, even when DN ORF50ΔSTAD was present, suggesting that apoptosis triggers KSHV replication through a distinct RTA-independent pathway. We verified that apoptosis triggers KSHV replication independent of RTA using ORF50 small interfering RNA (siRNA) and also showed that caspase activity is required to trigger KSHV replication. We showed that when apoptosis triggers KSHV replication, the kinetics of late gene expression is accelerated by 12 to 24 h and that virus produced following apoptosis has reduced infectivity. KSHV therefore appears to replicate via two distinct pathways, a conventional pathway requiring RTA, with slower replication kinetics, producing virus with higher infectivity, and an alternative apoptosis-triggered pathway that does not require RTA, has faster replication kinetics, and produces virus with lower infectivity. The existence of a distinct apoptosis-triggered, accelerated replication pathway may have evolutionary advantages for the virus and clinical significance for the treatment of KSHV-associated neoplasms. It also provides further evidence that KSHV can sense and react to its environment.
Figures
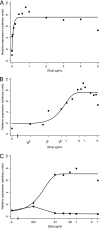
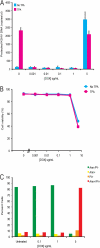
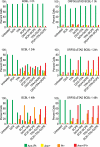
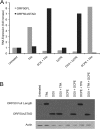
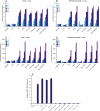
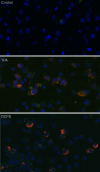
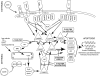
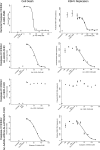
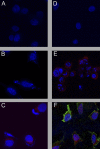
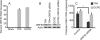
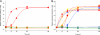
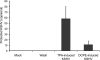
Similar articles
-
Identification of Novel Kaposi's Sarcoma-Associated Herpesvirus Orf50 Transcripts: Discovery of New RTA Isoforms with Variable Transactivation Potential.J Virol. 2016 Dec 16;91(1):e01434-16. doi: 10.1128/JVI.01434-16. Print 2017 Jan 1. J Virol. 2016. PMID: 27795414 Free PMC article.
-
ARID3B: a Novel Regulator of the Kaposi's Sarcoma-Associated Herpesvirus Lytic Cycle.J Virol. 2016 Sep 29;90(20):9543-55. doi: 10.1128/JVI.03262-15. Print 2016 Oct 15. J Virol. 2016. PMID: 27512077 Free PMC article.
-
Early activation of the Kaposi's sarcoma-associated herpesvirus RTA, RAP, and MTA promoters by the tetradecanoyl phorbol acetate-induced AP1 pathway.J Virol. 2004 Apr;78(8):4248-67. doi: 10.1128/jvi.78.8.4248-4267.2004. J Virol. 2004. PMID: 15047839 Free PMC article.
-
Epigenetic regulation of Kaposi's sarcoma-associated herpesvirus replication.Semin Cancer Biol. 2009 Jun;19(3):153-7. doi: 10.1016/j.semcancer.2009.02.010. Epub 2009 Feb 21. Semin Cancer Biol. 2009. PMID: 19429478 Free PMC article. Review.
-
Regulation of KSHV Latency and Lytic Reactivation.Viruses. 2020 Sep 17;12(9):1034. doi: 10.3390/v12091034. Viruses. 2020. PMID: 32957532 Free PMC article. Review.
Cited by
-
Establishment of Tree Shrew Animal Model for Kaposi's Sarcoma-Associated Herpesvirus (HHV-8) Infection.Front Microbiol. 2021 Sep 16;12:710067. doi: 10.3389/fmicb.2021.710067. eCollection 2021. Front Microbiol. 2021. PMID: 34603235 Free PMC article.
-
Apoptosis-induced activation of HIV-1 in latently infected cell lines.Retrovirology. 2015 May 16;12:42. doi: 10.1186/s12977-015-0169-1. Retrovirology. 2015. PMID: 25980942 Free PMC article.
-
Epstein-Barr Virus MicroRNA miR-BART20-5p Suppresses Lytic Induction by Inhibiting BAD-Mediated caspase-3-Dependent Apoptosis.J Virol. 2015 Nov 18;90(3):1359-68. doi: 10.1128/JVI.02794-15. Print 2016 Feb 1. J Virol. 2015. PMID: 26581978 Free PMC article.
-
Deregulation of KSHV latency conformation by ER-stress and caspase-dependent RAD21-cleavage.PLoS Pathog. 2017 Aug 30;13(8):e1006596. doi: 10.1371/journal.ppat.1006596. eCollection 2017 Aug. PLoS Pathog. 2017. PMID: 28854249 Free PMC article.
-
Emerging Proviral Roles of Caspases during Lytic Replication of Gammaherpesviruses.J Virol. 2018 Sep 12;92(19):e01011-17. doi: 10.1128/JVI.01011-17. Print 2018 Oct 1. J Virol. 2018. PMID: 30021896 Free PMC article. Review.
References
-
- Arvanitakis L, Geras-Raaka E, Varma A, Gershengorn MC, Cesarman E. 1997. Human herpesvirus KSHV encodes a constitutively active G-protein-coupled receptor linked to cell proliferation. Nature 385:347–350 - PubMed
-
- Bais C, et al. 1998. G-protein-coupled receptor of Kaposi's sarcoma-associated herpesvirus is a viral oncogene and angiogenesis activator. Nature 391:86–89 - PubMed
-
- Ballestas ME, Chatis PA, Kaye KM. 1999. Efficient persistence of extrachromosomal KSHV DNA mediated by latency-associated nuclear antigen. Science 284:641–644 - PubMed
-
- Boshoff C, et al. 1997. Angiogenic and HIV-inhibitory functions of KSHV-encoded chemokines. Science 278:290–295 - PubMed
Publication types
MeSH terms
Substances
Grants and funding
LinkOut - more resources
Full Text Sources
Other Literature Sources