Wnt signaling requires sequestration of glycogen synthase kinase 3 inside multivesicular endosomes
- PMID: 21183076
- PMCID: PMC3022472
- DOI: 10.1016/j.cell.2010.11.034
Wnt signaling requires sequestration of glycogen synthase kinase 3 inside multivesicular endosomes
Abstract
Canonical Wnt signaling requires inhibition of Glycogen Synthase Kinase 3 (GSK3) activity, but the molecular mechanism by which this is achieved remains unclear. Here, we report that Wnt signaling triggers the sequestration of GSK3 from the cytosol into multivesicular bodies (MVBs), so that this enzyme becomes separated from its many cytosolic substrates. Endocytosed Wnt colocalized with GSK3 in acidic vesicles positive for endosomal markers. After Wnt addition, endogenous GSK3 activity decreased in the cytosol, and GSK3 became protected from protease treatment inside membrane-bounded organelles. Cryoimmunoelectron microscopy showed that these corresponded to MVBs. Two proteins essential for MVB formation, HRS/Vps27 and Vps4, were required for Wnt signaling. The sequestration of GSK3 extended the half-life of many other proteins in addition to β-Catenin, including an artificial Wnt-regulated reporter protein containing GSK3 phosphorylation sites. We conclude that multivesicular endosomes are essential components of the Wnt signal-transduction pathway.
Copyright © 2010 Elsevier Inc. All rights reserved.
Figures
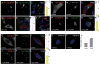
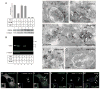
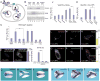
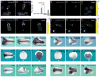
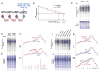
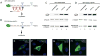
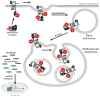
Comment in
-
Wnt signaling: multivesicular bodies hold GSK3 captive.Cell. 2010 Dec 23;143(7):1044-6. doi: 10.1016/j.cell.2010.12.003. Cell. 2010. PMID: 21183070
-
Membrane trafficking: a GSK3 lockdown.Nat Rev Mol Cell Biol. 2011 Feb;12(2):72. doi: 10.1038/nrm3052. Nat Rev Mol Cell Biol. 2011. PMID: 21252990 No abstract available.
Similar articles
-
Arginine methylation is required for canonical Wnt signaling and endolysosomal trafficking.Proc Natl Acad Sci U S A. 2018 Jun 5;115(23):E5317-E5325. doi: 10.1073/pnas.1804091115. Epub 2018 May 17. Proc Natl Acad Sci U S A. 2018. PMID: 29773710 Free PMC article.
-
Presenilin deficiency or lysosomal inhibition enhances Wnt signaling through relocalization of GSK3 to the late-endosomal compartment.Cell Rep. 2012 Nov 29;2(5):1316-28. doi: 10.1016/j.celrep.2012.09.026. Epub 2012 Nov 1. Cell Rep. 2012. PMID: 23122960 Free PMC article.
-
Multivesicular GSK3 sequestration upon Wnt signaling is controlled by p120-catenin/cadherin interaction with LRP5/6.Mol Cell. 2014 Feb 6;53(3):444-57. doi: 10.1016/j.molcel.2013.12.010. Epub 2014 Jan 9. Mol Cell. 2014. PMID: 24412065
-
Endocytic control of growth factor signalling: multivesicular bodies as signalling organelles.Nat Rev Mol Cell Biol. 2011 Nov 23;13(1):53-60. doi: 10.1038/nrm3244. Nat Rev Mol Cell Biol. 2011. PMID: 22108513 Free PMC article. Review.
-
Wnt, GSK3, and Macropinocytosis.Subcell Biochem. 2022;98:169-187. doi: 10.1007/978-3-030-94004-1_9. Subcell Biochem. 2022. PMID: 35378708 Review.
Cited by
-
Enhancement of β-catenin activity by BIG1 plus BIG2 via Arf activation and cAMP signals.Proc Natl Acad Sci U S A. 2016 May 24;113(21):5946-51. doi: 10.1073/pnas.1601918113. Epub 2016 May 9. Proc Natl Acad Sci U S A. 2016. PMID: 27162341 Free PMC article.
-
Epsin is required for Dishevelled stability and Wnt signalling activation in colon cancer development.Nat Commun. 2015 Mar 16;6:6380. doi: 10.1038/ncomms7380. Nat Commun. 2015. PMID: 25871009 Free PMC article.
-
PAG1 directs SRC-family kinase intracellular localization to mediate receptor tyrosine kinase-induced differentiation.Mol Biol Cell. 2020 Sep 15;31(20):2269-2282. doi: 10.1091/mbc.E20-02-0135. Epub 2020 Jul 29. Mol Biol Cell. 2020. PMID: 32726167 Free PMC article.
-
MicroRNAs in fluorosis pathogenesis: impact on dental, skeletal, and soft tissues.Arch Toxicol. 2024 Dec;98(12):3913-3932. doi: 10.1007/s00204-024-03853-9. Epub 2024 Sep 13. Arch Toxicol. 2024. PMID: 39269498 Review.
-
The complex world of WNT receptor signalling.Nat Rev Mol Cell Biol. 2012 Dec;13(12):767-79. doi: 10.1038/nrm3470. Epub 2012 Nov 15. Nat Rev Mol Cell Biol. 2012. PMID: 23151663 Review.
References
-
- Angers S, Moon RT. Proximal events in Wnt signal transduction. Nat Rev Mol Cell Biol. 2009;10:468–477. - PubMed
-
- Bilic J, Huang YL, Davidson G, Zimmermann T, Cruciat CM, Bienz M, Niehrs C. Wnt induces LRP6 Signalosomes and promotes Dishevelled-dependent LRP6 phosphorylation. Science. 2007;316:1619–1622. - PubMed
Publication types
MeSH terms
Substances
Grants and funding
LinkOut - more resources
Full Text Sources
Other Literature Sources
Research Materials